A previously unseen kind of radioactivity may explain why matter exists — including humans. A team of physicists launches an experiment to find an unusual phenomenon.
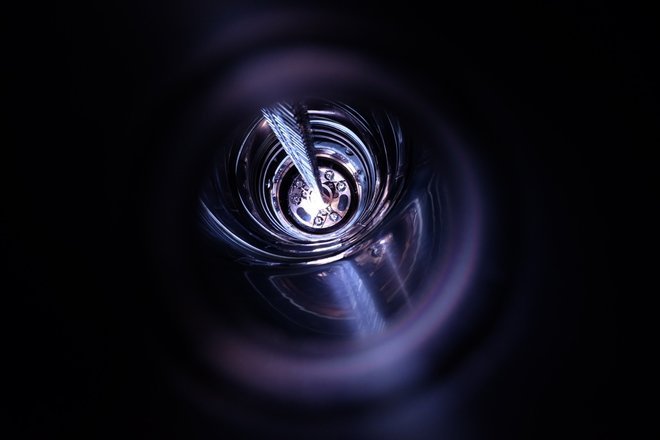
When the universe was formed about 13.7 billion years ago, according to modern theories, matter and its strange relative, antimatter, should have appeared in equal numbers during the Big Bang. Physicists know that when these two entities come into contact, they annihilate. But in that case there would be nothing in space except photons and neutrinos. And, nevertheless, we exist. Calculations show that matter turned out to be slightly more than antimatter - but why?
One way to explain this asymmetry is to find the difference between two types of matter, with the exception of charge, which can explain the advantage of ordinary matter. In modern physics, this is a very big question, because according to modern theories, they should behave the same way.
Strange neutrinos
In a study, physicists are trying to achieve
neutrinoless double beta decay . Usually, during beta decay, the unstable nucleus of a radioactive atom loses a neutron. The neutron turns into a proton, emitting an electron and a small particle, electron antineutrino. There is also a mirror situation in which a proton turns into a neutron, emitting a positron and an electron neutrino, the twin of the antineutrino. Double beta decay occurs when two electrons and two antineutrinos are emitted: in fact, beta decay occurs twice. Scientists have long been building a theory about the neutrino-free version of this process - in it neutrinos are annihilated before leaving the atom. In this case, the neutrino behaves like its own antiparticle.
')
Particles that behave like their own antiparticles are called Majorana fermions, in honor of the Italian physicist Ettora Majorana, who hypothesized this subject in 1937.
If neutrinos and antineutrinos behave differently, it can help explain why at the time of the formation of the Universe all matter did not annihilate.
Search for decay
But such an event is difficult to detect, because this is hampered by a strong “background noise”, as Bernhard Schwingenheuer, a representative of the Germanium Sensor Array project,
GERmanium Detector Array , or GERDA, says. The cause of the noise are cosmic rays.

The GERDA installation, hiding in an underground laboratory in Italy, consists of sensors contained in a bath with liquid argon enriched with the moderately radioactive isotope of germanium-76. Its half-life is 1.78 x 10
21 (1.78 billion trillion years) —that is how long it takes for half of its atoms to turn into selenium. This time is several orders longer than the existence of the Universe.
Typically, germanium in the process of its slow decay emits two electrons and two electron antineutrinos, which is the usual double beta decay. Physicists wanted to know whether such a decay occurs without neutrinos.
With such a long half-life, you might think that expecting such an event would take too long; but half-life is a probabilistic phenomenon. That is why the study uses about 38 kg of germanium mixed with liquid argon. This gives about 4.5 x 10
25 atoms, which means that several atoms should disintegrate right during the observations.
The GERDA team collected data for seven months, from December 2015 to June 2016. They did not detect decay, but were able to set a lower limit for the frequency of its occurrence: its half-life is 5.3 x 10
25 years, which means that the chance to see the decay of one atom during this time is 50%.
Extending the Standard Model
If decay is detected, it will mean that neutrinos are antiparticles to themselves, like photons. Otherwise, neutrino-free decay cannot take place. It also means that such radioactive decay is asymmetric. Recall that beta decay has a mirror version - electrons and antineutrinos, or positrons and neutrinos are emitted. If double beta decay is not symmetrical, it means that neutrinos and antineutrinos behave differently. This is not the case for other particle / antiparticle pairs.
This phenomenon will affect the Standard Model, which, although very successfully explains particle physics, is not complete. The model predicted the existence of the Higgs boson. But Schwingenhauer notes that there is evidence that neutrinos have a small mass (opened only in 1998, for which in 2015 the Nobel Prize was received), as well as signs of the presence of dark matter. All this suggests that the Standard Model did not become the last word of science.
"If neutrinoless double beta decay is detected, we can solve a couple of problems," says Philip Barbeau, assistant professor of physics at Duke University. “First, it will help to explain the asymmetry of matter-antimatter in the Universe. Secondly, it will help to understand why the neutrino has such a small mass. We can also estimate the neutrino mass, since the decay rate is related to the mass neutrino scale. ”
And then it remains to understand the physics of the process. The GERDA project has not yet demonstrated the desired decay, but this does not mean that it will not find it, as Schwingenhauer says. It is impossible to completely eliminate the probability of such a process, since there will always be the possibility that the time required for it to appear is simply more than was thought. Today, they set a lower limit for half-life, but future experiments may increase this value.
If, after numerous runs, they still do not see decay, then Barbo believes that this is unlikely to pave the way for new scientific models. “From the point of view of the underlying theories, we do not go back to the calculations. We simply will not know whether neutrino fermions are Majoranes ”.