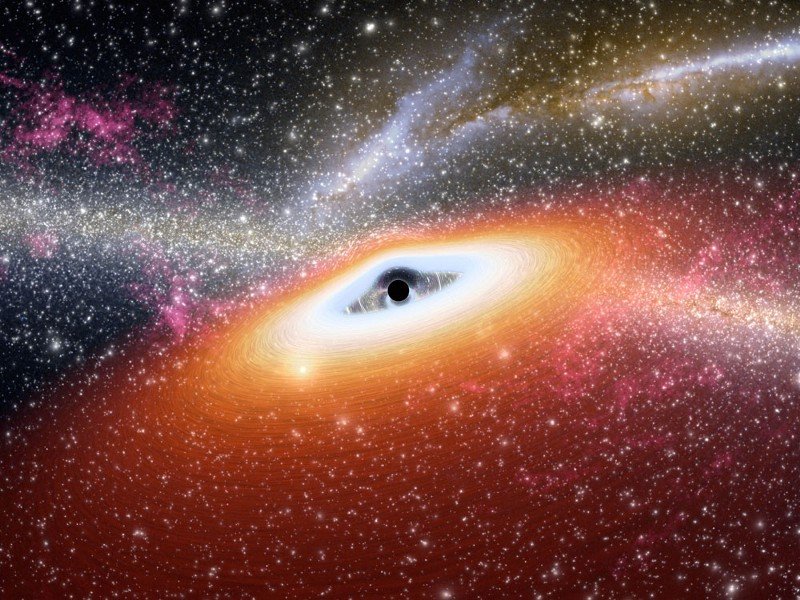
Black holes are one of the most extreme objects of the Universe: the concentration of mass is so huge that it collapses into a singularity in its center, in complete agreement with the general theory of relativity. Atoms, nuclei and even fundamental particles are crushed to an arbitrarily small thickness in our three-dimensional space. At the same time, everything that falls into it is doomed to never appear back, but simply to complement its gravitational pull. What does this mean for dark matter? Our reader asks:
How does dark matter interact with black holes? Does it suck in a singularity, like ordinary matter, and does it complement the black hole mass? If so, what happens to it after the black hole evaporates through Hawking radiation?
')
An excellent question, and you should start with what black holes are.

With us on Earth, in order to send something into space, it is necessary to overcome the gravitational pull of the Earth. On our planet, what we call the second cosmic velocity is 11.2 km / s, and it can be reached with the help of powerful rocket launches. If we were on the surface of the Sun, this speed would be almost 55 times greater, that is, equal to 617.5 km / s. After death, our Sun will shrink to a white dwarf, whose mass is approximately equal to 50% of the current mass of the Sun, and its size - to the size of the Earth. In this case, the second cosmic velocity on its surface will be equal to 4570 km / s, or 1.5% of the speed of light.

Sirius A and B, a normal star like the Sun and a white dwarf. Although the white dwarf is much smaller in mass, its small, earthly size makes the second cosmic velocity much larger.

This is important because the more mass you collect in one area of ​​space, the closer to the light speed needed to run away from this object. And when this speed on the surface of an object reaches or exceeds the speed of light, then it’s not just the light that cannot break out, it is already necessary - based on our understanding of matter, energy, space and time - everything inside the object collapses into a singularity. The reason is simple: all fundamental interactions, including the forces holding atoms, protons and even quarks, cannot move faster than light. So if you are somewhere outside the point of singularity and are trying to keep the object a little further away against the gravitational collapse, nothing will work out for you; collapse is inevitable. And to overcome this limitation, to begin with, you need a star that is more massive than 20-40 mass of the Sun.
A massive star at the end of its life, with its iron core falling through and forming a black hole.When its core runs out of fuel, the center will collapse inward under its own gravity, and create a catastrophic supernova, shedding and destroying the outer layers, and leaving a black hole in the center. These “stellar mass” BHs, whose mass is about 10 solar ones, grow with time, absorbing any matter or energy that dared to approach them too much. Even if you fall into the BH at the speed of light, you cannot get out. Due to the extremely strong curvature of the space inside, you will inevitably fall into the singularity in the center. After that, you just add the energy of BH.
Black hole absorbs accretion diskBeing outside it is impossible to say whether this BH was originally made from protons and electrons, neutrons, dark matter or even antimatter. A BH can measure, as far as we know, only three properties: mass, electric charge and angular momentum, that is, its rotational speed. Dark matter, as far as we know, has neither electric charge, nor quantum numbers (
color charge ,
baryon number ,
lepton number , etc.) that could be preserved or destroyed according to the paradox of the disappearance of information in BH.

Due to the principle of BH formation (due to explosions of supermassive stars), for the first time after their occurrence they are almost 100% composed of normal (baryonic) matter, and 0% of dark matter. Recall that dark matter interacts only through gravity, in contrast to normal matter, interacting through gravitational, weak, electromagnetic, and strong interactions. Yes, of course, dark matter in large galaxies and clusters is about five times more than normal, but if we sum it up with a giant galactic halo. In a typical galaxy, this halo extends over several million light years, spherically, in all directions, and normal matter is concentrated in a disk, with a volume of 0.01% of dark matter.
Normal matter in the central disk and dark matter in the blue halo of a typical galaxyBHs are usually formed inside a galaxy, where normal matter dominates dark matter. Consider the section of space where we and our Sun are located. If you circle it with a sphere with a radius of 100
AU. around the solar system, we will include all the planets, moons, asteroids and almost the entire Kuiper belt, but the baryonic mass — normal matter — of what is inside will be mainly represented by the mass of the Sun and will be about 2 * 10
30 kg. On the other hand, the total amount of dark matter in this area will be 1 * 10
19 kg, that is, about 0.0000000005% of the mass of normal matter in the same area, which is approximately equal to the mass of a modest asteroid like Juno, a small planet, about 200 km across.

Over time, dark matter and normal matter will collide with this black hole, and it will absorb them, adding to its mass. Most of the BH mass growth will be due to normal matter, not dark matter, although, at some point, quadrillion years later, the BH decay rate will still exceed its growth rate. Hawking radiation will emit particles and photons outside the black hole event horizon, retaining all the energy, charge, and angular momentum of the BH insides. This process can take from 10
67 years (for BHs with a mass from the Sun) to
10,100 years (for the most massive BHs, whose masses are billions of times more than the sun), but the result is a mixture of everything that is possible.

This means that BH will emit dark matter, but this does not depend at all on whether a particular BH had once absorbed dark matter. BH remembers that a substance that has fallen into it, only a small set of quantum numbers, and the amount of dark matter that has fallen into it is not included in this set. It comes out of it is not what was included!
An example of Hawking radiation leaving a BH from sites near the event horizon (just a quality illustration!)So, in the end, dark matter is just another source of food for BH, and is not very good. It is not even a particularly interesting source of food. The results of dark matter getting into BH would not differ from the results of an experiment in which you would shine a flashlight into BH, and it would absorb your photons. It is enough to pour into it, according to the equation E = mc
2 , as much energy as dark matter in terms of mass has fallen into it. There are no other charges in dark matter, and therefore, apart from the angular momentum acquired due to falling not at the center of the BH (which also applies to photons), it will not have any effect on the BH.