Detailed and simple description of the work of solar panels and forecasts for the future
How to drink tea in TibetOur
recent review of solar panels could have left you with the impression that collecting solar energy is new, but people have been exploiting it for thousands of years. With it, they heat the houses, prepare and heat the water. Some of the earliest documents describing the collection of solar energy go back to ancient Greece. Socrates himself said, “in the houses facing the south, the winter sun penetrates through the gallery, and in summer the path of the sun passes over our head and directly above the roof, because of which a shadow forms.” He describes how the Greek architecture used the dependence of the solar paths on the seasons.
In the V century BC. The Greeks faced an energy crisis. The predominant fuel, charcoal, ended as they cut down all the forests for cooking and heating. Wood and coal quotas were introduced, and olive groves had to be protected from citizens. The Greeks approached the problem of crisis, carefully planning the urban development, to ensure that each house can take advantage of the sunshine described by Socrates. The combination of technology and enlightened regulators worked, and the crisis was avoided.
Over time, the technology of collecting solar thermal energy only grew.
New England colonists borrowed house-building technology from the ancient Greeks to keep warm in cold winters. Simple passive solar water heaters, no more complicated than a black-painted barrel, were sold in the USA at the end of the 19th century. Since then, more sophisticated solar collectors have been developed, pumping water through panels that absorb or focus light. Hot water is stored in an insulated tank. In freezing climates, a two-fluid system is used in which the sun heats a mixture of water with antifreeze passing through a coil in a water storage tank, which performs another role, the role of a heat exchanger.
')
Solar collectors on the roofs of CyprusToday there are many complex commercial systems available for heating water and air in the house. Solar collectors are installed all over the world, and most of them in terms of per capita are in Austria, Cyprus and Israel.
Rooftop Solar Collector in Washington DCThe modern history of solar panels begins in 1954, with the discovery of a practical way of extracting electricity from light: Bell's laboratories discovered that it is possible to make photovoltaic material out of silicon. This discovery became the basis of today's solar panels (devices that turn light into electricity) and launched a new era of solar energy. Through intensive research, today's era of solar energy continues, and the sun intends to become the main source of energy in the future.
What is a solar cell?
The most common type of solar cell is a semiconductor device made of silicon - a distant relative of a solid-state diode. Solar panels are made from a set of solar cells connected to each other and creating a current output with the desired voltage and power. Elements are surrounded by a protective casing and covered with window glass.
Solar cells generate electricity due to the photovoltaic effect, not open in Bell laboratories. It was first discovered in 1839 by the French physicist Alexander Edmond Becquerel, the son of the physicist Antoine César Becquerel, and the father of the physicist Antoine Henri Becquerel, who received the Nobel Prize and discovered radioactivity. A little more than a hundred years later, in the Bell laboratory, a breakthrough was achieved in the manufacture of solar cells, which became the basis for creating the most common type of solar cells.
In the language of solid state physics, a solar cell is created on the basis of a pn junction in a silicon crystal. The transition is created by adding small amounts of different defects to different regions of the crystal; the interface between these areas will be the transition. On the n side, electrons are transferred, and on the p side, they are holes, where there are no electrons. In regions adjacent to the interface, the diffusion of charges creates internal potential. When a photon with sufficient energy enters the crystal, it can knock an electron out of an atom, and create a new electron-hole pair.
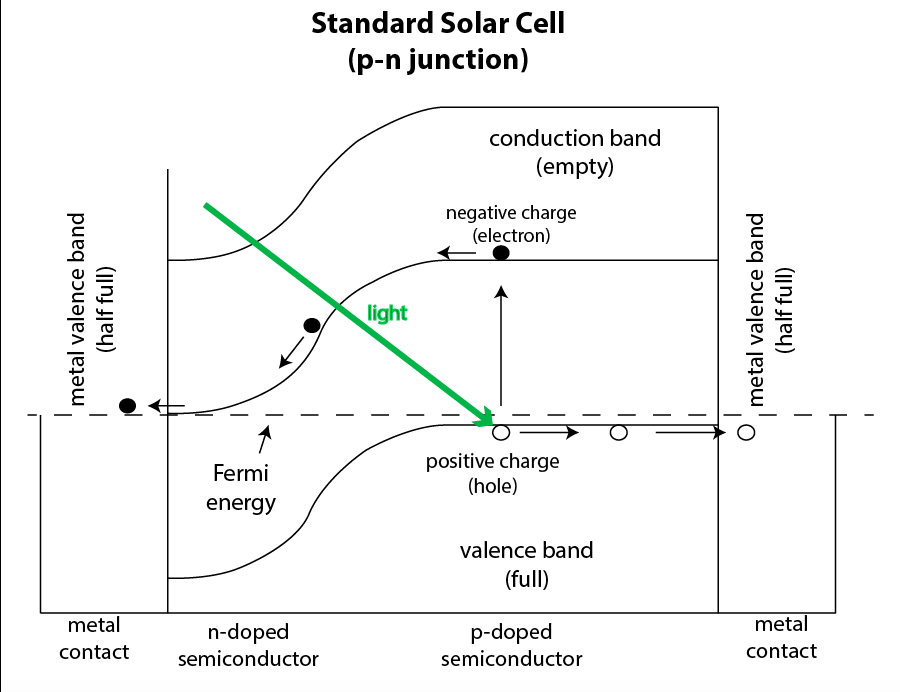
A newly released electron is attracted to holes on the other side of the transition, but because of the internal potential, it cannot transfer it. But if the electrons are provided with a path through the outer contour, they will follow it and light our homes along the way. Reaching the other side, they are recombined with holes. This process continues until the sun shines.
The energy required to release a bound electron is called the width of the forbidden band. This is the key to understanding why photovoltaic cells have an inherent efficiency limit. The width of the forbidden zone is a constant property of the crystal and its impurities. Impurities are regulated in such a way that, for a solar cell, the band gap is close to the photon energy from the visible spectrum. This choice is dictated by practical considerations, since the visible light is not absorbed by the atmosphere (in other words, as a result of evolution, people acquired the ability to see light with the most common wavelengths).
The photon energy is quantized. A photon with an energy less than the width of the forbidden band (for example, from the infrared part of the spectrum) cannot create a charge carrier. It will simply heat the panel. Two infrared photons will not work either, even if their total energy is enough. A photon of too much energy (say, from the ultraviolet range) will knock out an electron, but the excess energy will be wasted.
Since efficiency is defined as the amount of light energy falling on a panel divided by the amount of electricity produced - and since much of this energy is lost - efficiency cannot reach 100%.
The width of the forbidden zone for a silicon solar cell is 1.1 eV. As can be seen from the diagram of the electromagnetic spectrum, the visible spectrum is in the region slightly higher, so any visible light will give us electricity. But it also means that some of the energy of each absorbed photon is lost and converted into heat.
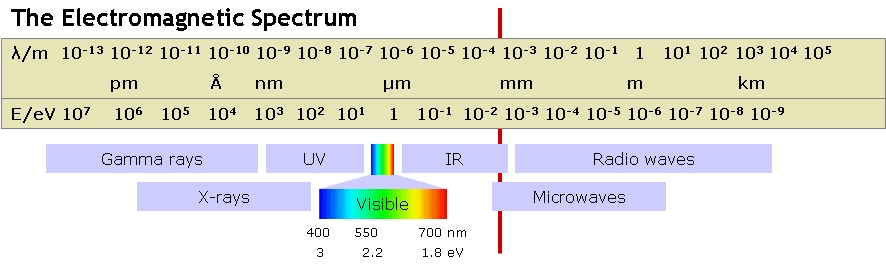
As a result, it turns out that even with an ideal solar panel, produced in flawless conditions, the theoretical maximum efficiency will be about 33%. In commercially available panels, the efficiency is usually 20%.
Perovskites
Most commercially installed solar panels are made from the silicon cells described above. But in laboratories all over the world, research is underway on other materials and technologies.
One of the most promising areas of recent times is the study of materials called perovskites. The
perovskite mineral, CaTiO
3 , was named in 1839 in honor of the Russian statesman Count
L. A. Perovsky (1792-1856) , who was a collector of minerals. The mineral can be found on any of the continents of the Earth and in the clouds of at least one exoplanet. Synthetic materials that have the same rhombic crystal structure as natural perovskite and have a chemical formula similar in structure are also called perovskites.

Depending on the elements, perovskites exhibit various useful properties, such as superconductivity, giant magnetoresistance, and photovoltaic properties. Their use in solar cells caused a lot of optimism, since their effectiveness in laboratory research has increased from 3.8% to 20.1% over the past 7 years. Rapid progress instils confidence in the future, especially as efficiency limits are becoming clearer.
In recent experiments at Los Alamos, it was shown that solar cells from certain perovskites approached silicon in efficiency, while being cheaper and easier to manufacture. The secret to the attractiveness of perovskites is the ability to easily and quickly grow millimeter-sized crystals without defects on a thin film. This is a very large size for an ideal crystal lattice, which, in turn, allows the electron to travel through the crystal without interference. This quality partially compensates for the non-ideal band gap of 1.4 eV, compared to the almost ideal value for silicon - 1.1 eV.
Most of the research aimed at increasing the efficiency of perovskites is related to the search for ways to eliminate defects in crystals. The ultimate goal is to make a whole layer for an element from an ideal crystal lattice. Researchers at MIT have recently made great progress on this issue. They discovered how to “heal” the defects of a film made from a particular perovskite, irradiating it with light. This method is much better than previous methods, including chemical baths or electric current, due to the absence of contact with the film.
Whether perovskites will lead to a revolution in the cost or effectiveness of solar panels is still unclear. It is easy to make them, but for now they are falling apart too quickly.
Many researchers are trying to solve the problem of decay. A joint study of the Chinese and the Swiss led to a new method of forming a cell from perovskite, free from the need to move holes. Since it is the layer with hole conductivity that degrades, the material must be much more stable.
Perovskite tin-based solar cellsA recent report from the Berkeley Lab describes how perovskites will one day be able to reach a theoretical efficiency limit of 31%, and still remain cheaper to manufacture than silicon ones. The researchers measured the conversion efficiency of various granular surfaces using atomic microscopy, measuring photoconductivity. They found that different facets are very different efficiency. Now researchers believe that they can find a way to produce a film on which only the most efficient edges will be connected to the electrodes. This can lead to a cell efficiency of 31%. If it works, it will be a revolutionary breakthrough in technology.
Other areas of research
It is possible to produce multi-layered panels, since the width of the forbidden zone can be adjusted by changing the additives. Each layer can be configured for a specific wavelength. Such cells can theoretically reach 40% efficiency, but so far remain expensive. As a result, they are easier to find on NASA satellite than on the roof of a house.
In a study by scientists from Oxford and the Institute of Silicon Photovoltaics in Berlin, layering was combined with perovskites. Working on the problem of material degradability, the team opened the possibility to create a perovskite with a custom width of the forbidden zone. They managed to make a cell version with a bandwidth of 1.74 eV, which is almost ideal for manufacturing together with a silicon layer. This can lead to the creation of low-cost cells with an efficiency of 30%.
A group from the University of Notreddam has developed photovoltaic paint from semiconductor nanoparticles. This material is not yet so effective as to replace solar panels, but it is easier to produce. Among the advantages - the possibility of applying to different surfaces. Potentially, it will be easier to use than rigid panels that need to be fixed to the roof.
A few years ago, a team from MIT made progress in creating solar thermal fuel. Such a substance can store solar energy within itself for a long time, and then issue it on request when using a catalyst or heating it. Fuel achieves this through the non-reactive transformation of its molecules. In response to solar radiation, molecules are converted into photo isomers: the chemical formula is the same, but the shape changes. Solar energy is stored as additional energy in the intermolecular bonds of the isomer, which can be thought of as a higher energy state of the original molecule. After starting the reaction, the molecules are transferred to the original state, transforming the stored energy into heat. Heat can be used directly or converted to electricity. This idea potentially eliminates the need to use batteries. Fuel can be transported and used energy somewhere else.
After publishing a work from MIT, in which
fulvalene diruthenia was used , some laboratories are trying to solve problems with the production and cost of materials, and to develop a system in which the fuel will be fairly stable in the charged state and able to “recharge” so that it can be used repeatedly. Just two years ago, the same scientists from MIT created solar fuel that could experience at least 2,000 charge / discharge cycles without any apparent deterioration in performance.
Innovation consisted in combining fuel (it was azobenzene) with carbon nanotubes. As a result, its molecules lined up in a certain way. The resulting fuel had an efficiency of 14%, and an energy density similar to a lead-acid battery.
Copper-zinc-tin sulphide nanoparticlesIn the newer works, solar fuel was produced in the form of transparent films that can be stuck on the windshield of the car. At night, the films melt the ice due to the energy gained during the day. The speed of progress in this area leaves no doubt that solar fuel will soon be transferred from the laboratories to the area of ​​familiar technologies.
Another way to create fuel directly from sunlight (artificial photosynthesis) is being developed by researchers at the University of Illinois at Chicago. Their “artificial leaves” use sunlight to convert atmospheric carbon dioxide into “synthesis gas” into a mixture of hydrogen and carbon monoxide. Synthesis gas can be burned or converted to more conventional fuels. The process helps remove excess CO
2 from the atmosphere.
The Stanford team created a prototype solar cell using carbon nanotubes and fullerenes instead of silicon. Their efficiency is much lower than commercial panels, but only carbon is used to create them. There are no toxic materials in the prototype. This is a more environmentally friendly alternative to silicon, but to achieve economic benefits, it needs to work on efficiency.
Research and other materials and production technologies. One of the promising areas of research includes monolayers, materials with a layer of one molecule thick (like graphene). Although the absolute photovoltaic efficiency of such materials is small, their efficiency per unit mass exceeds the usual silicon panels thousands of times.
Other researchers are trying to make solar cells with an intermediate range. The idea is to create a material with a nanostructure or a special alloy in which photons can work with energy insufficient to overcome the usual width of the forbidden zone. In such a material, a pair of low-energy photons can knock out an electron, which cannot be achieved in conventional solid-state devices. Potentially, such devices will be more efficient, as they use a larger range of wavelengths.
The variety of areas where photovoltaic cells and materials are explored, and the fast confident progress since the invention of the silicon cell in 1954, gives us confidence that the enthusiasm of solar energy will not only continue, but will also increase.
And these studies are happening just in time. In a recent meta-study, it was shown that solar energy overtook oil and gas in terms of the ratio of the received energy to the expended energy, or in terms of energy efficiency. This is a significant turning point.
There is little doubt that as a result, solar energy will turn into a significant, if not dominant, form of energy, both in industry and in the private sector. It remains to hope that the reduction in the need for burning fossil fuels will happen before an irreversible change in the global climate occurs.