Reading the
recent news about the next unprecedented breakthrough in the development of energy storage devices (charging a smartphone battery in just seconds, 30,000 charge / discharge cycles without loss of capacity, and most importantly, the capacity is ten times more than the existing models of this type of storage devices, ie supercapacitors and at least as good as lithium batteries) I, of course, did not believe it and went to read the original source, i.e. A
Nanowire Supercapacitor High-Performance Scientific
-1-Body Core Enabled by Conformal Growth of Capacitive 2D WS2 Layers published in ACS Nano.
Having run through her eyes and making sure that skepticism was reasonable and correct, at first I decided to add a couple of comments under the article and already become a duty officer on the GT picture and limit it to this:
But, after reading the article in its entirety, I decided that it nevertheless fully deserves attention, despite the fairness of the comic book on KDPV. There is something that is breakthrough and very promising in it. And what more (breakthroughs or "scientists cured cancer") I propose to evaluate the readers on their own after becoming acquainted with the real parameters of the invention under the cat. At the same time, there is an answer to some questions arising from readers, in particular, to what extent these “nanotechnologies” are suitable for industrial implementation (production) or this will remain nothing more than an interesting laboratory experiment.
Good news team
What is this new energy storage device? In fact, it is not new, it belongs to a class of long-known and widely used in practice supercapacitors (
ionistors ). But scientists managed to significantly improve their parameters.
')
One of the main problems in the development of ionistors is that it is necessary to simultaneously solve two problems that conflict with each other:
- increase the active area of ​​the electrodes and their ionic conductivity in order to increase the specific capacity
- increase the electronic conductivity of the electrodes in order to increase the power density and reduce energy losses during fast charging / discharging of the drive
In the previously existing classical technologies, the buildup of one parameter led to a significant deterioration of the other. Recent years have seen developments with the use of nanotechnology, which rather well combined both requirements, but they faced another problem - the rapid degradation of the formed nanostructures in the process of operation and the rapid loss of capacity. Some samples in this regard were inferior even to ordinary chemical batteries (they withstood only a few hundred cycles).
A breakthrough in this work can be called the fact that scientists managed
to climb on the Christmas tree and achieve high performance in three parameters simultaneously: specific capacity, specific power, resource.
How did you manage to achieve this? The basis of everything is the transition metal foil (in this case tungsten was used), which provides high performance in the second parameter (high electronic conductivity of the electrodes, and, consequently, high power density and low losses during charging / discharging).
Further, on the surface of this foil, a dense “forest” of nanowires (pins) of metal oxide coated with
chalcogenides of the same metal is formed (in this case
, tungsten sulfide - WS
2 ). Schematically it looks like this:
Brown on the diagram indicates pure metal, a lighter shade - its oxide, and blue - chalcogenide. The foil below is shown conditionally - in fact, its thickness is much larger (comparable or even greater than the height of the “pins”).
And this is the real nanotechnology, without quotes. This is how these pins (or as the scientists themselves called nanowires themselves) look under an electron microscope:
Above is a general view of the surface, with a “forest” of nanowires grown on it. (a) - a general view of a separate "wiring" on the side, (b) and (c) - a view of the surface (enlarged fragment in a red rectangle) on which a film of hackolgenide formed over the metal oxide is clearly visible, (d) is a cross section of a single wiring, ( f) and (e) - enlarged fragments marked blue and green.
Such a nanostructure allows for good performance in the first parameter: a large area of ​​the active surface of the electrodes that come into contact with the electrolyte, and, consequently, a high specific capacitance by the measurements of supercapacitors.
And high stability is achieved by the production method - these nanowires are not sprayed onto the surface, but literally grown from it, forming a single crystal structure with it, which gives them high strength / stability during operation. In the following graph (e), the test results of the obtained samples:
Even after 30,000 cycles, charge + discharge at high power (about 8 seconds for charging and 16 seconds for a full cycle) capacity remains higher than the original. And the first few thousand cycles, it even grows due to surface activation (growth of the active area of ​​the electrodes). The maximum is about 125% of the nominal capacity when operating at high currents (charge in ~ 8 seconds), more than 150% of the nominal capacity when operating at low currents (charge in ~ 160 seconds) and is reached after 2500 operating cycles.
Such "endurance" is far superior to any samples of "metal" ionistors (which have comparable specific power) and is at the level of the best classical carbon (which are inferior in specific power and capacity).
So, taking into account everything written above, we can say that the ideal energy storage device that everyone is waiting for is finally invented?
Physics, you heartless ...
Unfortunately not. As with all ionistors, this energy storage device has one very big drawback - a low specific capacity. In terms of capacity, a miracle is not allowed by the fundamental physical limitations. This is all the same ionistor that saves energy through the use of a
double electric layer and with appropriate restrictions.
In particular, the resulting elements have a very low operating voltage range: only from 0 to 0.8 Volts, which is even lower than typical levels for ionistors manufactured using other technologies. And the energy stored by any capacitors, as is known, depends on the square of the maximum voltage.
Electrical capacity (in Faradah), here, though significantly (at times) is higher than most analogs, but not by orders of magnitude, which are needed to compensate for lower operating voltage compared to other ionistors, and then also to catch up with chemical batteries, from which typical ionistors specific capacity lags dozens of times. The following graph shows the range of operating voltages and the dependence of the specific capacitance (milifarad per 1 cm
2 of the electrode area) on the charge / discharge rate:
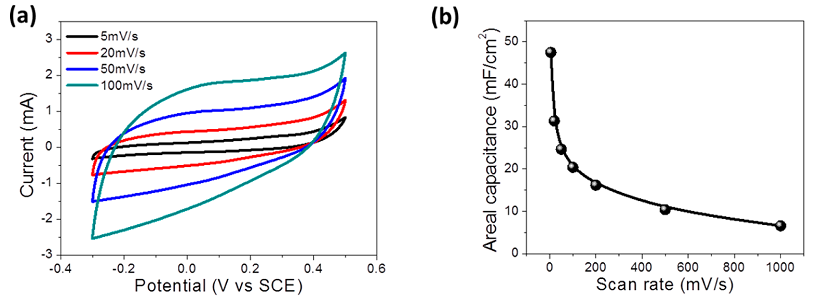
With such parameters, in order to at least equal to the long-standing and mass-produced lithium batteries, which have already become the standard, you just need to have a huge area of ​​electrodes and pack them very compactly.
For comparison, you can take a lithium battery of standard size 18650 (a cylinder with a diameter of 18mm, a length of 65mm and a weight of no more than 50 grams). The typical achieved capacity of such elements is now about 3 A * h, with an average operating voltage of 3.7 V, which gives about 40,000 J of stored energy per element: 3 * 3,600 * 3.7 = 39,960 J.
A supercondenser with an operating voltage of 0.8 V for storing the same energy will need a capacity of 40,000 / 0.8
2 * 2 = 125,000 Farad.
For such a capacity, the area of ​​each electrode is 125,000 / 0.05 = 2,500,000 cm
2 = 250 m
2 .
If you try to fit all this area of ​​electrodes into a similar small volume of 18650 elements, you will get a tape of more than 4000 meters long for each of the 2 electrodes and the same separator length between them. Such packing density is not even close to reach in practice. Moreover, it is not achievable even in theory (if we take the thickness of the base on which the “forest” is grown, equal to zero and equal to zero the distance between the electrodes, the resulting volume will be much larger than the battery).
Actually, the scientists who conducted the study understand this perfectly. They estimated the specific capacitance of capacitors when using similar electrodes at a level of about 0.06 Wh / cm
3 or 216 J / cm
3 . This is the most optimistic estimate (limit) is not achievable in practice, because only the volume of the electrodes themselves is taken into account without everything else (separator, electrolyte, housing). In practice, a good achievement will be the capacity 1.5-2 times lower than this limit.
By the standards of supercapacitors, these are simply chic parameters, which in the aggregate (capacity / power) are superior to all analogues. Below on the graph is a comparison with all already used in practice and considered as promising alternative capacitor production technologies (the discussed option is a set of black dots):

X axis - power density, Watt / cm
3Y-axis - specific capacity, Watt * hour / cm
3It is worth paying attention to the fact that both scales are logarithmic, one big division = a 10-fold increase in the parameter
But, compared with chemical batteries, the capacity is still very small. If we recall the same serial 18650 element based on lithium, then when the physical volume is less than 17 cm
3 and the stored energy is about 40,000 J, its specific capacity is about 2350 J / cm
3 . Those. at least 10 times higher than the maximum (theoretical) capacity of this development. And 15-20 times higher than can be expected from it in practice in the case of the start of production. By weight (j / kg) lag from lithium batteries will be even higher. According to the mass specific capacity, scientists did not provide data in their work, but it is clear that such elements will be heavy: the finished element at the macro level will be the most densely rolled roll of tungsten foil impregnated with electrolyte.
In general, you can immediately forget about batteries for smartphones, tablets and other electronics that charge in seconds. As well as about batteries for electric vehicles with charging in minutes and a huge resource. These are all pure inventions of journalists unrelated to reality (see KDPV). For such applications, the capacity is indecently small.
But in a number of segments, taking into account their characteristics (high power density and a very large resource, with not the worst capacity), such drives can be very promising.
For example:
- Electric storage buffer (hybrids or electric vehicles with a small capacity of the main battery, other electric vehicles such as electric jets) for regenerative braking and / or short-term afterburning, i.e. protection of the main battery from peak loads or a buffer for electric transport without a main battery at all (fuel cells).
- Intermediate high-power uninterruptible power supply (picks up the load when the main power fails, until the standby generator starts).
- Regulation of voltage surges and frequencies in power systems, which becomes every year more and more urgent as the widespread introduction of unstable renewable energy sources in power systems.
And other similar applications requiring high power density and frequent inclusions.
Soar or not?
The practical implementation of this technology will depend on the possibility of transfer from the laboratory to industrial production and, of course, on prices. If there is nothing definite to say about prices, the prospects for mass production look good. Unlike most other nanotechnology, risking never to leave the walls of the laboratory. The work describes in some detail the method of production of electrodes, and I have not found something particularly difficult to repeat in industry.
The general production scheme is presented in this figure:

Stages of production of electrodes:
- The base is taken thin tungsten foil (W). The surface is thoroughly cleaned of all impurities (in its work, it was “bathed” successively in acetone, hydrochloric acid solution, ethanol and finally washed with deionized water). The cleaned surface is covered with a 10% alkali solution (KOH).
- The prepared foil is placed in an oven and gently heated to 650 degrees, then kept for 2 hours at this temperature. In this process, the very “forest” of tungsten oxide nanowires (WO 3 ) is formed on the foil surface. After cooling, the foil is removed from the oven, washed with water from alkali residues and dried.
- At the last stage, the foil is placed in a chamber in which a low pressure and circulation of inert gas is maintained (argon was used in this experiment). In this chamber, it is heated to a high temperature (850 degrees) in the presence of a tank with purified sulfur (S). This is a simple variant of the CVD process ( Chemical deposition from the gas phase ) in which sulfur vapors react with tungsten oxide on the surface of nanowires and form a film of tungsten sulfide (WS 2 ) with a thickness of only a few nanometers. After 40 minutes of exposure in such conditions, the electrodes are cooled and they are ready for use.
Actually it is all technology. 2 sheets of such foil are placed in the electrolyte and form a supercapacitor. Many different substances are suitable as electrolytes: in particular, scientists tested solutions of sulfuric acid, lithium chloride, and potassium chloride. But the main part of the tests was carried out in a solution of
sodium sulfate (Na
2 SO
4 ).
There are no serious obstacles to mastering technology in industry. But, most likely, it will turn out to be quite expensive in production and in this regard there will also be a significant loss with respect to chemical batteries, the production of which is much simpler.
For a snack, a small survey. Now, when all the details are known without a spoiled phone, what do you think is more here?