As cosmic microwave background radiation — the residual glow of the Big Bang — continues to shed light on the birth of our Universe.
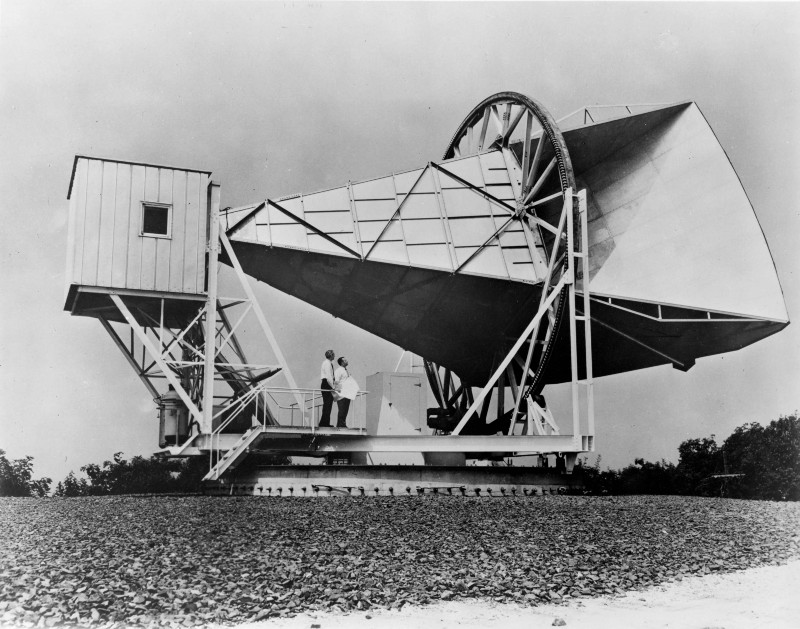
The announcement of the results of BICEP2, which showed the first evidence that gravitational waves could appear in the early Universe, fueled interest in cosmology among scientists and all the rest. Gravitational waves can polarize KMFI, the residual glow of the Big Bang, in a certain way, and it is the polarization signal that was detected by BICEP2 located at the South Pole. But the most recent data came from the Planck telescope, and judging by it, most of the BICEP2 results can be explained not by gravitational waves, but by the nearby dust covering the CMMF that we observe.
We need to wait for additional data, both from the joint work of BICEP2 and Planck, and from other experiments, in order to determine what proportion of cosmic dust related to gravitational waves took. One thing is clear: academic blogs and news sites will follow new discoveries. This text is an attempt to help the authors of future articles on new research in the CMPI-cosmology to get into context, starting with the foundations of the CMPI, how it was formed and what it can tell us. The main topic of the article will be the intensity of the CMBI (we call it temperature), and in the next article I will talk more about polarization.
Story
The first discovery of KMFI in 1964 happened by chance. Arnaud Penzias and Robert Wilson worked on an experiment in the Bell laboratory, and used balloons as reflectors to transfer communications in the microwave range between two points. To do this, they needed to know if any background noise would affect their measurements. They processed almost all the noise, except one: 2.73 K homogeneous microwave radiation, which, as it turned out, appeared 380,000 years after the Big Bang.
')
Since the discovery (for which scientists received the Nobel Prize in Physics in 1978), several experiments on Earth and in space have measured the CMBI with ever increasing accuracy. In 1992, the Cosmic Background Explorer (CoBE) experiment conducted the first observations of irregularity of radiation — small temperature changes, 100,000 times lower than the average background temperature of 2.73 K. Then, Wilkinson Microwave Anisotropy Probe (WMAP) expanded our knowledge of irregularities temperatures in 2003, and in 2013 Planck gave the most accurate measurement to date. New experiments not only increase the accuracy of temperature measurements, but also reduce the angular size of errors.

What is KMFI?
Before the formation of the CMBR, the usual components of the Universe were light (photons), hydrogen and helium nuclei, and free electrons. (Yes, there were still neutrinos and dark matter, but about them another time). Since free electrons are negatively charged, they interact with photons in the so-called Thomson scattering. If a photon and an electron intersect, they bounce off each other like billiard balls. At that time, photons had a very high energy, and the average temperature of the Universe was more than 3000 K. Such a temperature kept electrons in a free state, since the photon energy exceeded the ionization energy of atoms: the energy required for ejecting an electron from an atom. Instead of remaining attached to the positively charged nuclei of hydrogen and helium, the electrons were almost immediately knocked out by photons.
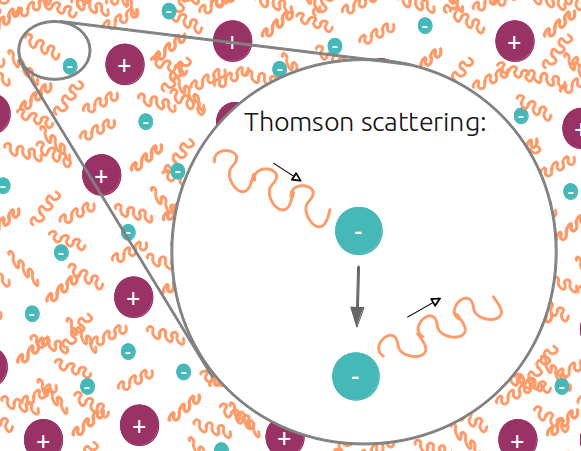
These two effects, photons involved in the ionization of atoms, and photons interacting with electrons, lead to important consequences. The high frequency of interactions means that the photon will not fly far before it collides with the electron again and changes direction. Imagine driving in a thick fog, when the headlights in front of a vehicle in front are not visible, as light scatters on water molecules. It was the same in the Universe before the appearance of KMFI - the light was completely absorbed by the mist of free electrons (they often speak of an opaque Universe). The combination of opacity and Thomson scattering gives the CMBR uniform temperature in all directions.

It is also known that there should be small fluctuations in the area of the uniform temperature of the CMBI, since the high frequency of interactions indicates the presence of photons everywhere where matter is present. You may have heard that the CMBR gives us information about the dark matter content in the Universe, or that the cold and hot spots of the CMBI correspond to more or less dense regions - and here's why. Dark matter does not interact with ordinary matter, so it can gather in dense bunches, while photons are trapped by a mist of free electrons. The gravitational attraction of dark matter bunches brings together nuclei and electrons, and with them the photons.
The fluctuations in the temperature of the photons that we observe in the CMPH directly tell us where the matter was located 13 billion years ago. (And if it doesn’t impress you that cosmologists were able to register KMFI, know that the observed temperature fluctuations are 100,000 times less than 2.73 K - these are microkelvins already!)

At the same time, space expanded and photon waves stretched. The photon energy is related to its wavelength, and the greater the length, the lower the energy. In the end, the expansion stretches the photons so much that their energy falls below the ionization energy. And at this moment electrons are combined with nuclei and get neutral hydrogen and helium (and some other things), and photons get the possibility of unimpeded distribution.

The moment of formation of neutral atoms is called recombination, and is often described as the transformation of the Universe into a transparent one. The photons, having escaped beyond the limits of the electronic fog, can travel in the direction in which they eventually meet the Earth and our KMFI sensors! The brief moment between the scattering of photons and the formation of neutral atoms is called the surface of the last scattering. It is him that shows us the KMFI. Since the Universe was opaque up to this point, we literally cannot see anything.
So what about these cards?
The best way to extract information from the KMFI’s distribution maps is to calculate the energy spectrum, and you must have seen one of them in popular science articles. The connection between hot and cold areas can be confusing, but in reality everything is very simple.
To understand this connection, we turn to a simple wave form. Any non-periodic smooth wave that you can find or draw has one important mathematical property: it can be written as the sum of many different periodic waves with specific frequencies and amplitudes. The wave can be described in real space, that is, it can be built on the x and y axes. But it can also be described in terms of a harmonic function, that is, to construct the dependence of the frequencies to be summed on the amplitudes of each of them. On the gif, the connection between the wave, how it can be broken down into a sum of different frequencies, and how it all relates to the harmonic function is available. For people with a mathematical education, you can simply say that this is a Fourier transform.
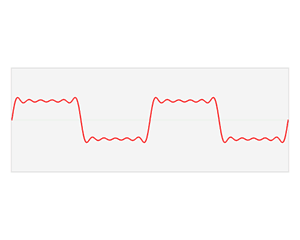
If, instead of waves on a plane, we imagine waves on the surface, this will be the CMBR - a pattern of hot spots (peaks) and cold spots (dips) located on the surface of the last scattering. Instead of a single image of temperature fluctuations of the KMFI, you can write them as a sum of different patterns, each of which corresponds to a particular mode or multipole.

The graphs of the power spectrum show how strong each mode is, and after they are summed up, they reproduce the picture of the CMBR.

The brilliant idea of the power spectrum in cosmology is that we can make predictions about its form based on our understanding of the universe. The standard model for cosmology is called LambdaCDM, that is, Lambda (dark energy) Cold Dark Matter, and for most multipoles it remarkably coincides with the temperature spectrum of the CMBR. In the smallest multipoles there are some oddities, many of which are well described here.

So far, only the temperature of the observed CMFR has been discussed, but the photons also have polarization. Since light is an electromagnetic wave, it has intensity and orientation relative to the base coordinate system. The orientation direction is polarization, and the reason why dark glasses block glare so well. They filter light waves oriented in the same direction, usually reflected from a flat surface. The polarization of the KMFI (which is of two types, E-mode and B-mode), is decomposed into a power spectrum in the same way as temperature fluctuations.
These spectra add even more information about our early Universe, perhaps even be able to find evidence of the existence of prehistoric gravitational waves. But can they? It is this conflict between Planck and BICEP2 that scientists are trying to resolve!