Our Standard Model of Elementary Particles and Interactions has not so long become as complete as it could be desired. Every single elementary particle - in all their possible forms - was created in the laboratory, measured, and properties were determined for all. The longest keeping upper quark, antiquark, tau neutrino and antineutrino, and finally the Higgs boson, have fallen victim to our capabilities.
And the last one - the Higgs boson - also solved the old problem of physics: finally, we can demonstrate from where the elementary particles take their mass!
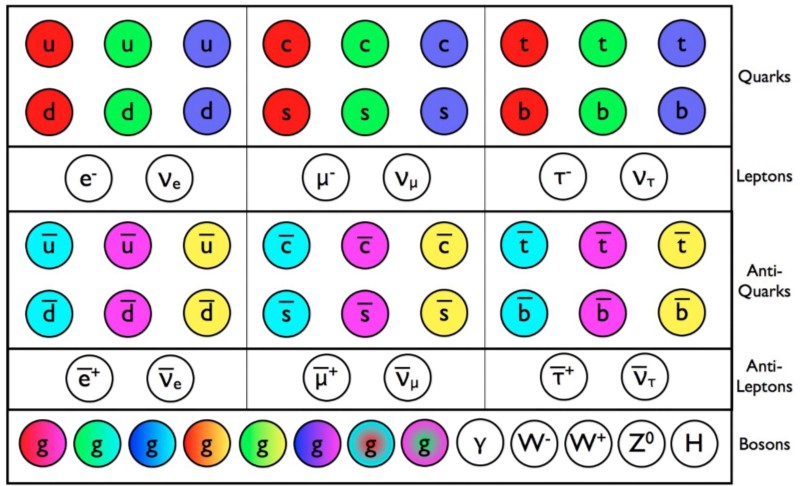
')
This is all cool, but science does not end at the moment when the solution of this riddle ends. On the contrary, it raises important questions, and one of them is “what next?”. As for the Standard Model, we can say that we still do not know everything. And for most physicists, one of the questions is especially important - to describe it, let's first consider the following property of the Standard Model.

On the one hand, weak, electromagnetic and strong interactions can be very important, depending on their energies and distances at which the interaction takes place. But with gravity, it's not like that.
If suddenly you read
this beautiful book of the author
Lisa Randal , she wrote a lot about this riddle, which I would call the greatest unsolved problem of theoretical physics: about the
problem of hierarchy .

We can take any two elementary particles - of any mass and subject to any interactions - and find that gravity is 40 orders of magnitude weaker than any other force in the Universe. This means that the force of gravity is 10
40 times weaker than the three remaining forces. For example, although they are not fundamental, but if you take two protons and scatter them a meter, the electromagnetic repulsion between them will be 10 to
40 times stronger than gravitational attraction. Or, in other words, we need to increase the force of gravity by 10,000,000,000,000,000,000,000,000,000,000,000,000 times in order to level it with any other force.
In this case, it is impossible to simply increase the mass of the proton by 10
20 times, so that gravity pulls them together, overcoming the electromagnetic force.

Instead, in order for reactions like the one illustrated above to occur spontaneously, when protons overcome their electromagnetic repulsion, you need to bring together 10
56 protons. Only by gathering together and succumbing to the force of gravity, can they overcome electromagnetism. It turns out that 10
56 protons will make up the minimum possible mass of the star.
This is a description of how the universe works - but why it is so, we do not know. Why is gravity so weaker than other interactions? Why is the "gravitational charge" (ie, mass) so weaker than the electric or color, or even weak?
This is the problem of hierarchy, and for many reasons it is the greatest unsolved problem of physics. The answer is unknown to us, but we can not say that we are in complete ignorance.
Theoretically , we have some good ideas about finding a solution, and a tool for finding evidence of their correctness.

So far, the Large Hadron Collider - the highest energy collider - has reached unprecedented energy levels in the laboratory, collected a bunch of data, and reconstructed what was happening at the collision points. This includes the creation of new, hitherto unseen particles (such as the Higgs boson), and the appearance of old, well-known particles of the Standard Model (quarks, leptons, gauge bosons). He is also capable, in the case of their existence, to produce any other particles that are not part of the Standard Model.
There are four possible methods known to me - that is, four good ideas - solving the problem of hierarchy. The good news is that if nature chose one of them, then the LHC will find it! (And if not, the search will continue).

In addition to the Higgs boson found several years ago, no new fundamental particles were found at the LHC. (Moreover, there are no intriguing new candidates for particles at all). And yet, the found particle fully corresponded to the description of the Standard Model; there were no statistically significant hints at the new physicist. Neither the composite Higgs bosons, nor the multiple Higgs particles, nor non-standard decays, nothing like that.
But now we started receiving data from even higher energies, twice the previous ones, up to 13-14 TeV, in order to find something else. And what, in this key, are possible and reasonable solutions to the problem of hierarchy?

1) Supersymmetry, or SUSY. Supersymmetry is a special symmetry capable of making the normal masses of any particles large enough to allow gravity to be comparable with other influences, mutually annihilate with a high degree of accuracy. This symmetry also assumes that each particle in the standard model has a partner superparticle, and that there are
five Higgs particles and five of their superpartners. If such symmetry exists, it must be broken, or the superpartners would have the same mass as ordinary particles, and they would have been found long ago.
If SUSY exists on a scale suitable for solving the problem of hierarchy, then the LHC, having reached energies of 14 TeV, must find at least one superpartner, as well as the second Higgs particle. Otherwise, the existence of very heavy superpartners will in itself lead to another problem of the hierarchy, which does not have a good solution. (Interestingly, the absence of SUSY particles at all energies will refute string theory, since supersymmetry is a necessary condition for string theories containing the standard model of elementary particles).
Here is the first possible solution to the problem of hierarchy, which currently has no evidence.

2) Technicolor. No, it is not a color cinema system from the 1950s, it is a physical term for designating theories that require new gauge interactions, and either not having Higgs particles, or having unstable or unobservable (i.e., composite) Higgs particles. If technicolor were confirmed, he would also need a
new and interesting set of observed particles. In principle, this system could be a solution to our problem, but the recent discovery of a spin zero particle at the required energy level seems to refute this possible solution. Now, if this Higgs particle turned out to be not a fundamental, but a composite one, made of several fundamental ones, this would help the theory to remain an acceptable solution. Future checks on the LHC on energies of 13-14 TeV will be enough to find out for sure.
There are two more possibilities, one of them is more promising, but both of them include additional dimensions.

3) Rolled up extra dimensions. This theory, introduced by the already mentioned Lisa Randal [Lisa Randall] with Raman Sundrum [Raman Sundrum], postulates that gravity is in fact just as strong as the other influences, but not in the Universe with three spatial dimensions. It lives in another Universe with three spatial dimensions, shifted relative to ours by only 10
-31 meters in the fourth spatial dimension (or, as shown in the diagram above, in the fifth dimension, when time is turned on). This theory is interesting because such a system would be stable and could offer an explanation of why the Universe expanded so quickly at the very beginning (and the convoluted space-time is capable of such), therefore it has strong advantages.
It should also include an additional set of particles; not supersymmetric, but Kaluza-Klein particles, and this is due to the presence of additional dimensions. By the way, in the space experiment
a hint was made of the existence of Kaluza-Klein particles at energies of 600 GeV, or with a mass 5 times greater than that of Higgs. And, although such energies are not yet attained at current colliders, the new LHC should be able to create such particles in abundance, if they exist.

But the existence of this new particle is not guaranteed, since the signal received is only an excess of the electrons observed compared with the expected background. But it must be borne in mind, because when the LHC accelerates to maximum energies, almost all particles with a mass below 1000 GeV will have to be within its reach.
And finally ...

4) Large extra dimensions. Additional measurements may not be coagulated, but large, but larger only in comparison with coiled ones, the size of which is
10-31 m. “Large” measurements must be millimeter in size, therefore new particles must begin to appear within the limits of the LHC capabilities. Again, Kaluza-Klein particles can appear, and this may be a possible solution to the hierarchy problem.
But one consequence of this model is that gravity will strongly deviate from Newtonian at distances less than a millimeter, and it is very difficult to verify this. Modern experimenters, however, are
ready to accept the challenge .

It is possible to create tiny supercooled brackets filled with piezoelectric crystals (which produce electricity during deformation), with distances between them of the
order of microns . This technology allows us to impose restrictions on 5-10 microns on “large” measurements. In other words, gravity works according to the predictions of GR on scales much smaller than a millimeter. So if there are large additional dimensions, they are at energy levels inaccessible to the LHC, and more importantly, do not solve the hierarchy problem.
Of course, a
completely different solution may be found for the hierarchy problem, which cannot be found at modern colliders, or there is no solution at all; it may simply be a property of nature without any explanation for it. But science will not advance without trying, and this is exactly what these ideas and searches are trying to do: to advance our knowledge of the universe forward. And, as always, with the start of the second launch of the LHC, I look forward to what may appear there, except for the already discovered Higgs boson!