If our "standard candles" are not very standard, will there be dark energy?
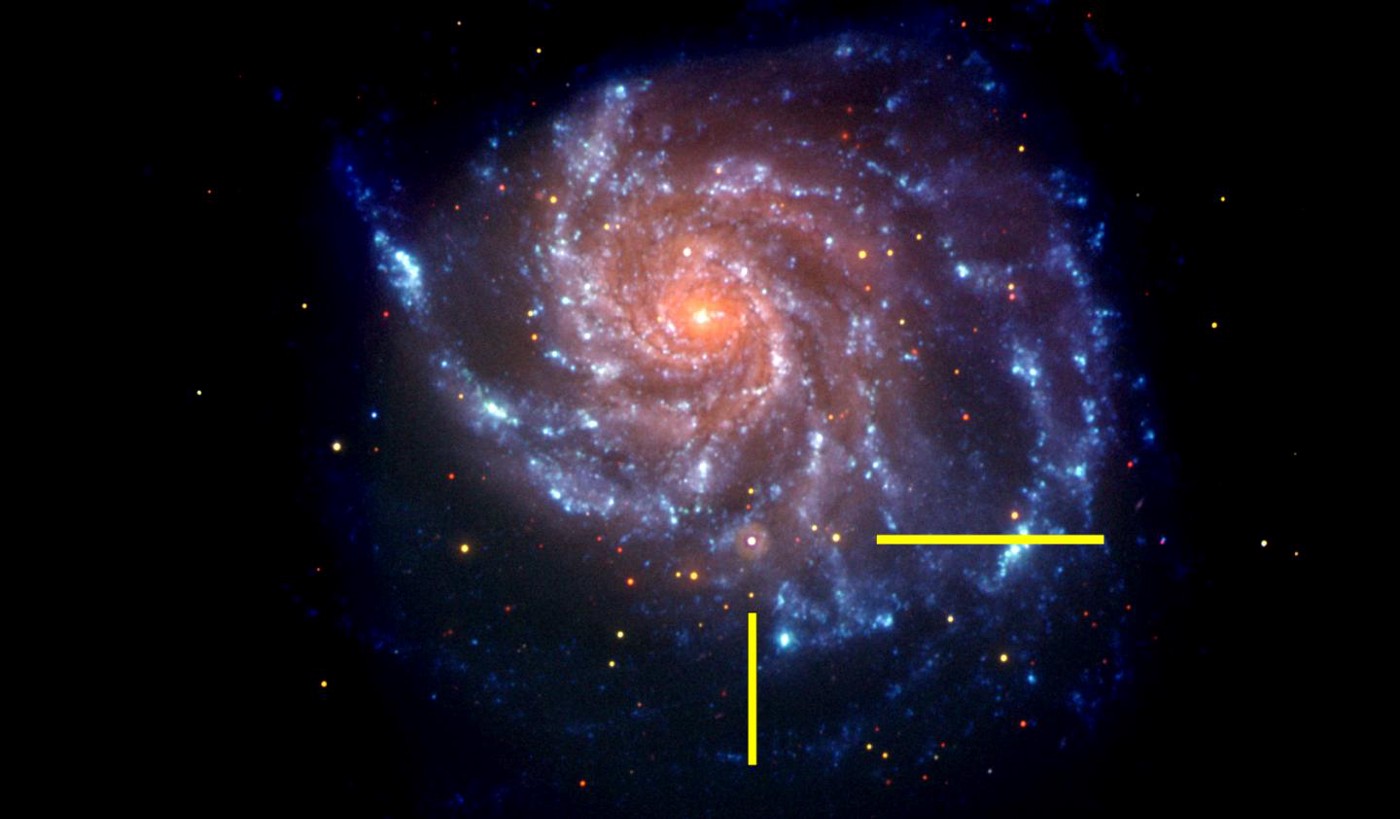
Alone: "The world will end in fire!"
- “No, - will destroy the ice!”
Kohl passion fervor is known to me
I would prefer to burn in the fire.
But if twice death awaits,
How fragile the world is, I can understand
Knowing the hate ice:
To break the world
And the ice will come down.
- Robert Frost
From time to time, amazing discoveries occur in science that permanently change our understanding of the universe. In the late 1990s, observations of distant supernovae clearly showed that the Universe is not just expanding, but that distant galaxies increase the speed of escape from us. This discovery, awarded the Nobel Prize, told us about the fate of our universe. But among your questions this week there is a question from João Carlos, who mentioned the new study and asked a question about it:
I read an article on Eurekalert here and thought that you, too, need to read it. Can't wait for your comments about her.
')
The article was written according to a press release from the University of Arizona - I was there a postdoc just a few years ago. It states the following:
The university team found that the type of supernovae commonly used to measure distances in the Universe is divided into previously unknown categories.This is potentially a very serious factor affecting our understanding of the universe and its fate. Let's go back 100 years ago to the lesson we had to learn, and then move back to today to see why.

In 1923, Edwin Hubble examined the incomprehensible, dim "spiral nebula" in the sky, studying the supernovae appearing in them and trying to add to the treasury of our knowledge the essence of these objects. Some argued that these were embryos of stars in the Milky Way, others believed that they were “island universes”, separated by millions of light years from our Galaxy, consisting of billions of stars.
Watching the big nebula in Andromeda on October 6 of that year, he saw an explosion of a new star, then a second, and then a third. And then something unprecedented happened: the fourth new one appeared on the spot of the first one.
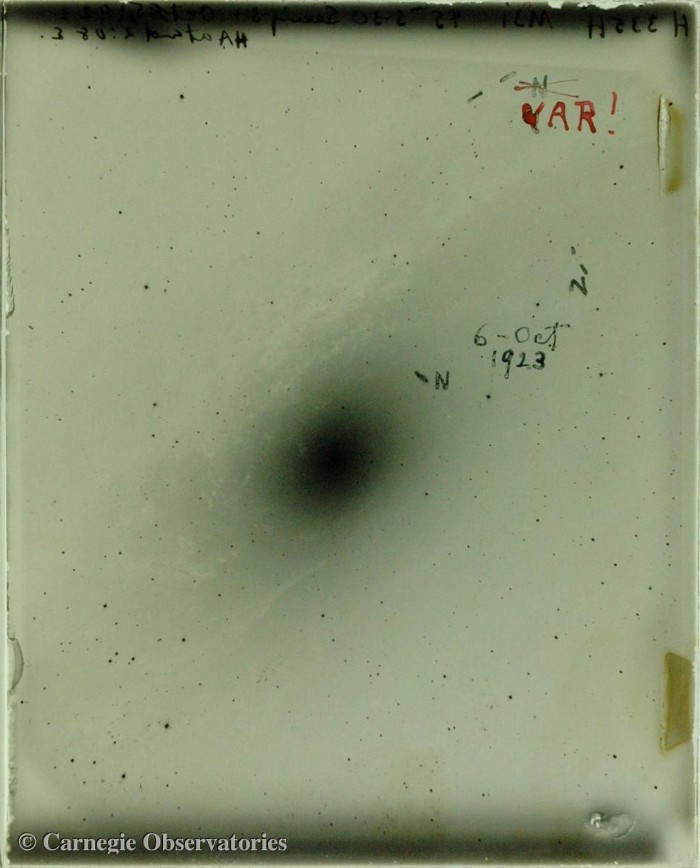
Explosions of new stars sometimes happen again, but for this they usually need hundreds and thousands of years, because this happens when enough fuel accumulates on the surface of a collapsed star in an amount sufficient to ignite. Of all the new ones that we have discovered, even the fastest stars re-ignition takes many years. The idea of rerunning a new one for several hours was absurd.
But there was an object known to us that could go from a bright to a dim state and back in a few hours: a variable star! (Therefore, he crossed out the letter “N”, denoting a new one, and wrote “VAR” - a variable).
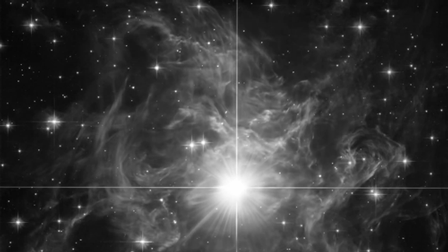

The incredible work of Henrietta Swan Leavitt taught us that some stars in the Universe — Cepheids, pulsating variable stars — go from bright to dim with a certain period, and this period is inseparably linked to their true brightness. This is important because if you measure the period (which is easy), you will know the true brightness of the star. And since you can easily measure the apparent brightness, you will immediately know the distance to the object, since the ratio of brightness to range has been known for hundreds of years!

Hubble used this information about variable stars and the fact that we can find them in these spiral nebulae (now known as galaxies) to measure their distance from us. He compared their known redshift with distances and derived the Hubble law and the rate of expansion of the Universe.
Cool, yeah? But we, unfortunately, often present this discovery in a too favorable light: Hubble’s conclusions about the rate of expansion of the Universe were wrong!

The problem was that the Cepheid variables measured by Hubble in these galaxies differed from the Cepheids measured by Henriette Levitt. It turned out that Cepheids are divided into two classes, which Hubble did not know about. And although Hubble’s law worked, his initial estimations of distances were too small, and therefore his assumptions about the rate of expansion of the universe turned out to be too large. Over time, we corrected them, and although the general conclusions — that the Universe is expanding, and that spiral nebulae are galaxies distant from ours — have not changed, the details of expansion have definitely changed!
Fast forward to today.

Supernovae shine brighter than Cepheid, and can often surpass in brightness — albeit for a short time — their entire galaxy! Instead of millions of light years, they can be seen, under favorable circumstances, at distances of more than ten billion light years, which allows us to look further and further into the Universe. In addition, a special type of supernovae, Ia, is obtained from an out-of-control fusion reaction inside white dwarfs.
When such reactions occur, the entire star is destroyed, but what is important, the light curve of the supernova, that is, how it becomes brighter with time, and then dimmer, is well known, and has universal properties.

By the end of the 1990s, enough data was collected on supernovae located at sufficiently large distances, and two independent teams — the Supernova Search Team High-z [High-z Supernova Search Team] and the Cosmological Project on Supernova [Supernova Cosmology Project] - announced that the acceleration of the expansion of the Universe follows from these data, and that some form of dark energy dominates in the Universe.
Like many others, I was skeptical, because if supernovae were not as well studied as we think, all these conclusions would have to be discarded.
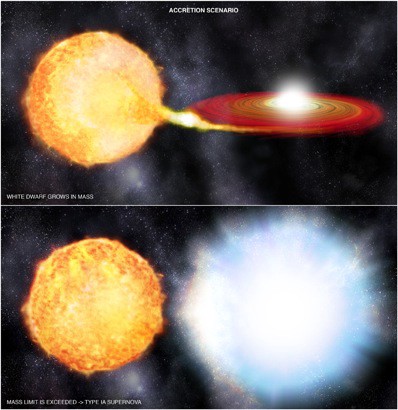
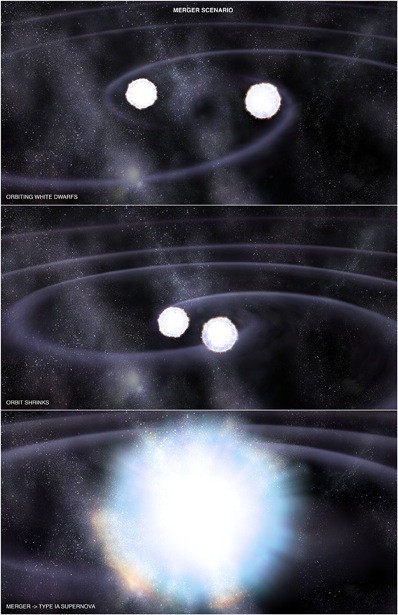
First, supernovae can appear in two different ways: from the accretion of matter from the companion star (L) and from merging with another white dwarf ®. Will these paths lead to the appearance of identical supernovae?
Secondly, these supernovae, removed over great distances, may appear in conditions that are very different from those that are close to us. Is it certain that the light curves we see correspond to light curves at great distances?
Thirdly, it is possible that something happened to this light while he was making his amazing journey for a gigantic distance. Is there really no new type of dust or some kind of property that mutes the light (for example, photon-axion oscillations)?
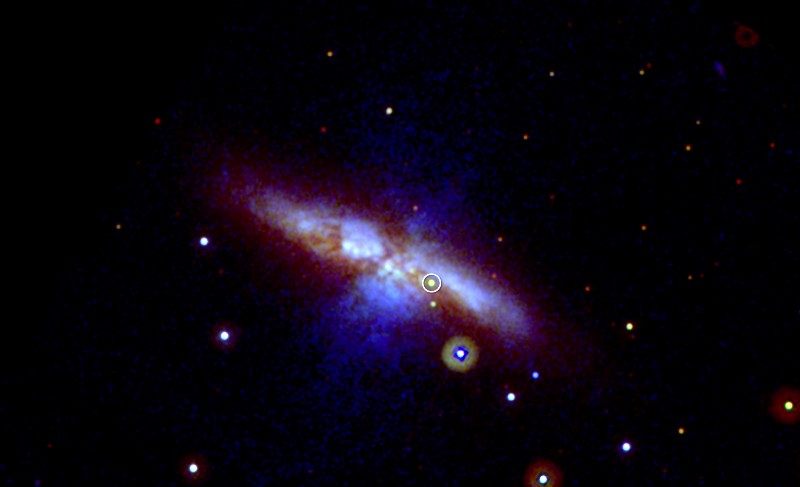
It turns out that all these questions can be resolved and dismissed. They are not problems. But recently, by approaching the heart of the question of João Carlos, we discovered that these so-called. “Standard candles” may not be so standard. Just as Cepheids are of different types, these type Ia supernovae are also of different types.
Imagine that you have a box of candles that you thought were identical: they could be lit, put at different distances, and immediately, by measuring the apparent brightness, find out how far they are. This idea is used in astronomy, and therefore type Ia supernovae are so necessary to us.
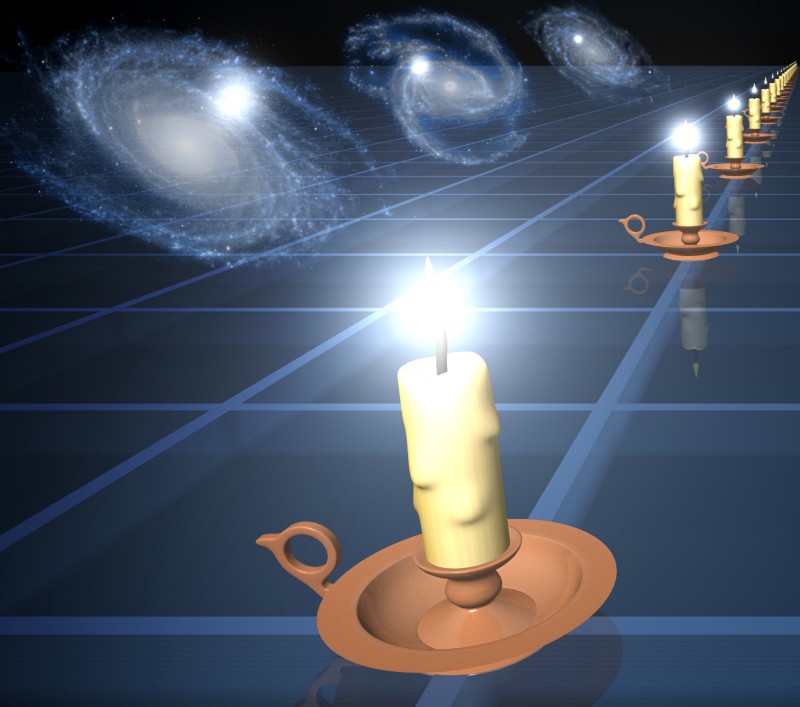
Now imagine that the flame of these candles has a different brightness! Suddenly, some are a little brighter or a little dimmer; You have two types of candles, and brighter ones may be closer to you, and dimmer ones may be further.
This is exactly what we have discovered among the supernovae: there are two different classes, and one is slightly brighter in the blue / ultraviolet range, and the other is brighter in the red / infrared, and their light curves are different. This may mean that at redshifts (long distances), the supernovae themselves are just dimmer, and not further away from us.
In other words, our conclusion about the acceleration of the expansion of the Universe can be based on incorrect interpretation of the data!
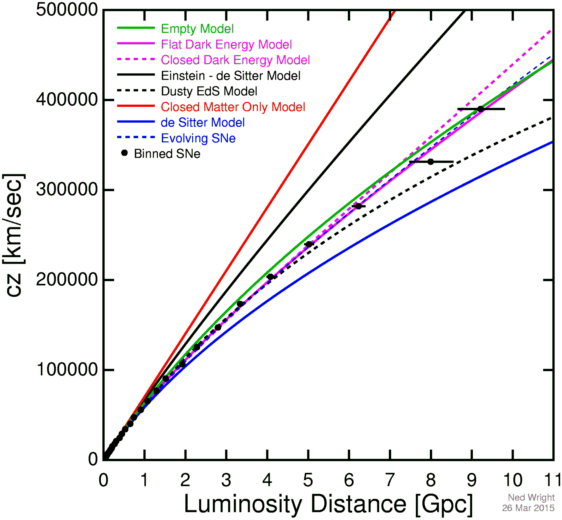
If we incorrectly measured the distances to these supernovae, maybe we were wrong about dark energy! At least in this regard, we would be very worried. We would not worry so much if dark energy had not gone anywhere, but it would have been less than previously thought.
So which of these reasons for anxiety are real? It turns out that only a reason for not much excitement. In 1998, only supernova data spoke in favor of dark energy. Over time, we collected two more equally strong and independent proofs.
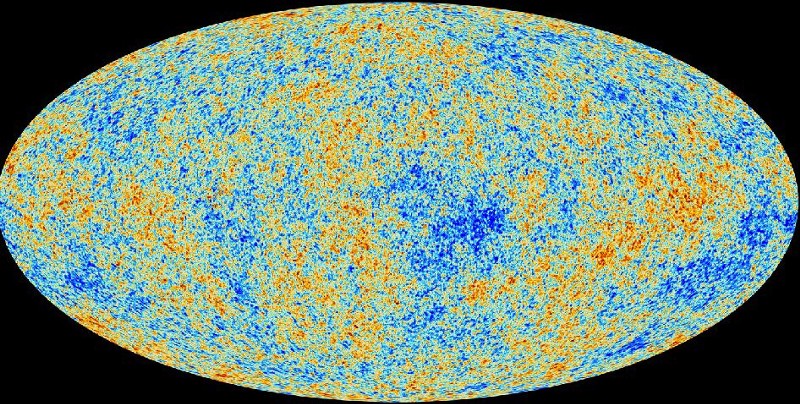
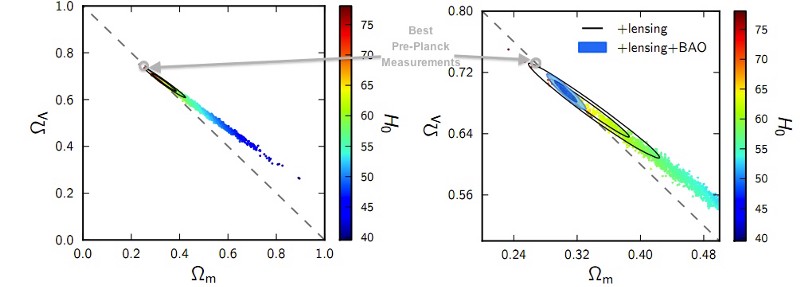
1) Cosmic microwave background radiation. Fluctuations in the residual glow from the Big Bang - measured by the WMAP project, and then with greater accuracy and by the Plank project - clearly indicate that the Universe contains 5% of normal matter, 27% of dark matter and 68% of dark energy. And although microwave radiation in itself does not speak about the properties of dark energy, it says that 2/3 of the energy of the Universe is in a form that does not crumple and does not belong to the mass.
For a while this was a problem, since the supernovae themselves said that dark energy is 3/4 from the Universe, so perhaps new discoveries on supernovae will help us to better build the data.

2) The way in which galaxies gather in clusters. In the early Universe, dark matter and normal matter — and how they interact, or not interact, with radiation — controlled how galaxies had gathered to the present moment in clusters. If you look at any galaxy in the Universe, you will find a strange property in it: another galaxy is more likely to be at a distance of 500 million light years from it, and with a smaller one - at a distance of 400 or 600 million years. This phenomenon is known as baryon acoustic oscillations [Baryon Acoustic Oscillations, BAO], and is due to the fact that normal matter is under radiation pressure, but dark matter is not.
The thing is that the Universe is expanding because of everything that is present in it, including dark energy. Therefore, with the expansion of the universe, this preferred distance of 500 million light years is changing. Instead of “standard candles”, BAO allows us to use a “standard ruler”, which can also be used to measure dark energy.
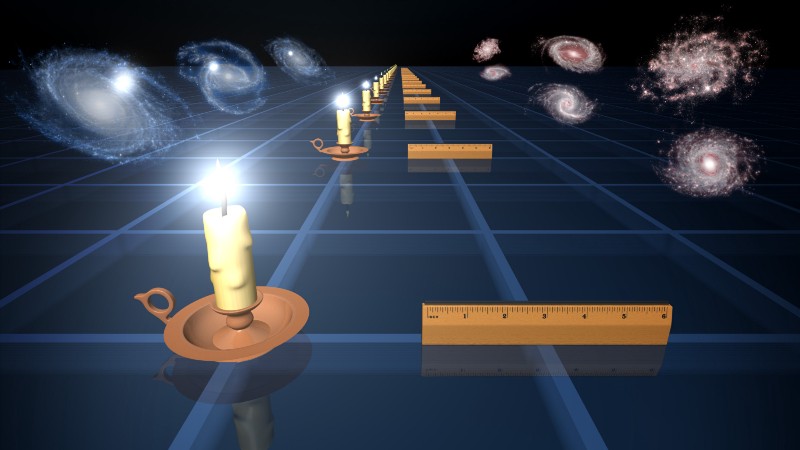
It turns out that measurements made through BAO are not worse than measurements made on supernovae and give the same results: The universe consists of 70% of dark energy and corresponds to the presence of a cosmological constant, not the walls of domains, cosmic strings or other exotic theories .
If we combine all three data sets, we find that they give us a roughly similar picture.
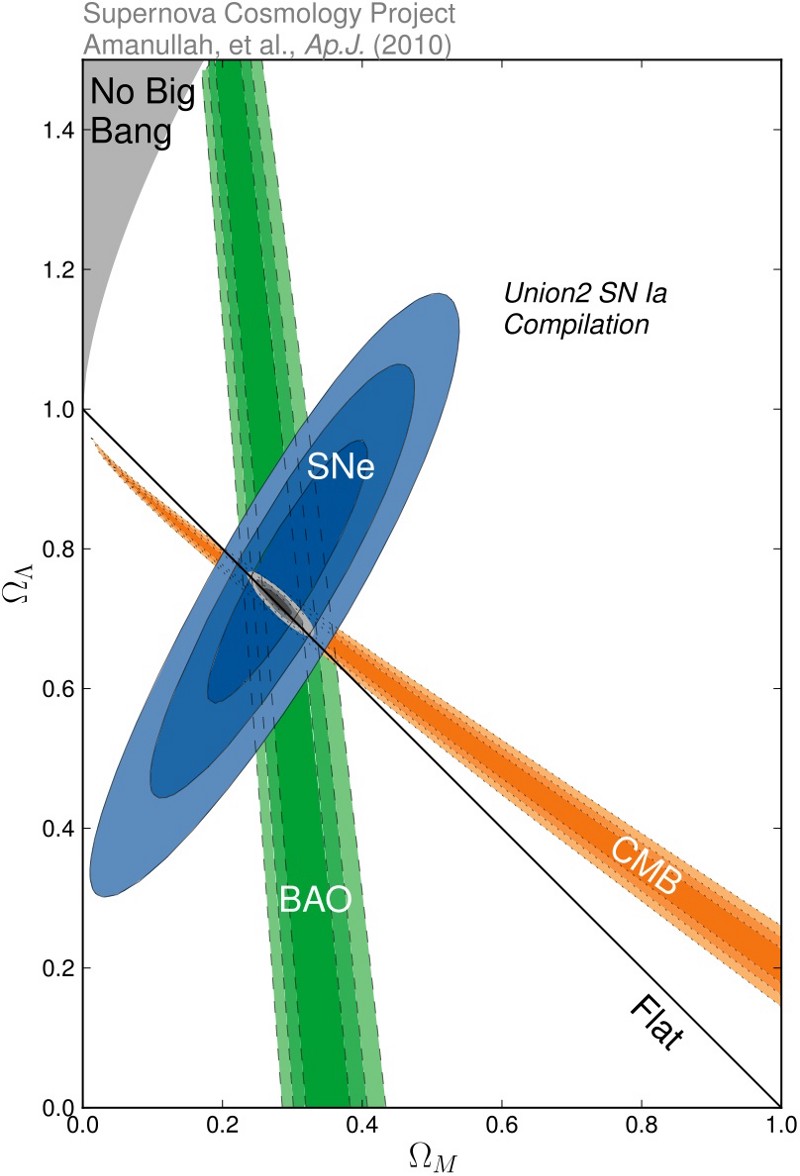
What we have learned is that the amount of dark energy and its type, which we derive from supernovae, may slightly change, and this may even help us bring the three indicated methods - supernovae, CMFI and BAO - in the best fit. This is one of those great moments of science, when one wrong assumption does not force us to throw out all the results and conclusions, but it helps us to more accurately understand the phenomenon that has plunged us into confusion since its discovery.
Dark energy exists, and thanks to a new discovery, we can even understand it - and its effect on the Universe - better than before. Thanks to the reader for the opportunity to talk about such an interesting discovery.
Send me your questions and suggestions for the following articles.