As a feature based on the observation of cosmic inflation, it can proclaim the scientific revolution of the century (March 18, 2014)
Despite the name, the Big Bang Theory is not a blast theory at all. This is a theory of the consequences of an explosion.
- Alan Guth
When you imagine the beginning of the Universe, you probably think of a hot, dense state filled with matter and radiation, which expands and cools incredibly quickly (and, by the way, that was how it was). But what cannot be done is to extrapolate back to an arbitrarily hot and dense state. You may think that without problems you will pass back through time, to a “singularity” with infinite temperature and density, when all the energy of the Universe has been compressed into a single point - but this is not true.
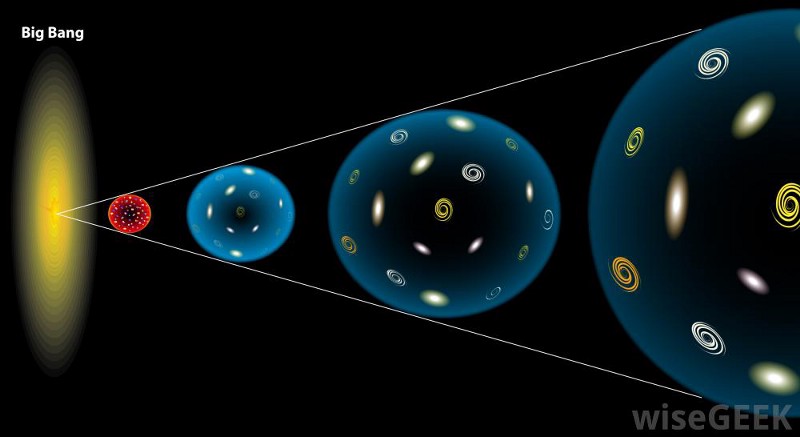
')
One of the remarkable features of the universe is that the radiation that originated at that time still exists. It underwent reflections from charged particles during the time of the Universe, which was young, hot, and ionized (and this lasted for 380,000 years). When the Universe became electrically neutral (when matter first formed neutral atoms), the radiation remaining from the Big Bang rushed in a straight line, not interrupted by this neutral matter.

As the universe expanded — due to the fact that the radiation energy is determined by the wavelength — these wavelengths stretched along with the expansion of space, and the energy has since dropped very strongly. But it helps us a lot, as it provides material for observations.

And if we could see and measure these waves, they would give us a window to look into the early Universe! And so, in the 1960s, Arno Penzias and Robert Wilson discovered this residual luminescence from the Big Bang - radiation, evenly going in all directions, only a few degrees above absolute zero - and in it scientists immediately recognized the microwave cosmic background radiation, which have been looking for so long!

After 50 years, we have made incredible progress. We were able not only to measure the energy spectrum of this radiation, but also to measure the tiny temperature fluctuations inherent in it, as well as their scale, their relationship with each other and how it all relates to the evolution of the Universe.
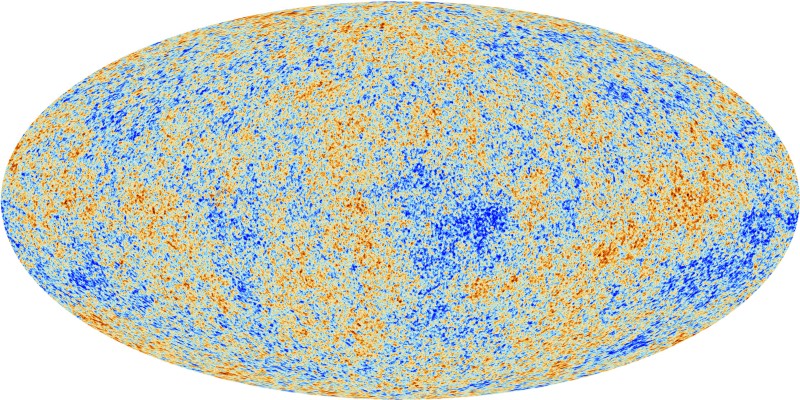

In particular, we learned how the universe looked at 380,000 years old, what it was made of, and how the interacting matter affected the radiation on its way to our eyes, 13.8 billion years long.
But there is something else that can give us information about these things: we can study not only the energy and temperature of light, but also its polarization. Let me explain.

In fact, light is an electromagnetic wave. So, it consists of oscillating electric and magnetic fields perpendicular to each other, it has a special wavelength (determined by energy), and it propagates at the speed of light.
Flying past charged particles, reflecting off the surface, interacting with other electromagnetic phenomena, electric and magnetic fields react with their environment.

Initially, the resulting light must be unpolarized, but a huge amount of things lead to its polarization in a variety of ways. In other words, light, which usually has randomly oriented electric and magnetic fields, can experience interactions, as a result of which they will have a preferred orientation. And now she will be able to tell us a lot of cognitive things about that, with whom the light interacted during its history.

The polarization effect of background microwave radiation was first discovered in the past decade with the help of the WMAP satellite, and even better results are expected from the Planck Observatory in the future (but this type of research, it should be noted, is very difficult to implement). Polarization, due to which the light looks “radial”, is called the E-mode of polarization (for electric fields), and the one due to which the light is “twisted” is called the B-mode of polarization (for magnetic fields).

Most of the effects observed were due to the billions of light years of matter that passed through the light; we call this "foreground." He had to go all the way in all directions since the era of radiation to reach our eyes today.

But a tiny, tiny part of the polarization had to reach us from earlier times. You see, before the Big Bang - before the Universe could be described as hot, dense, and filled with matter and radiation - the Universe simply expanded exponentially; it was a period of cosmic inflation. At this time, the energy inherent in the Universe itself was inherent in the most empty space - energy in quantities much larger than is present in it today.

At this time, quantum fluctuations — intrinsic to the space itself — stretched across the universe, and provided the initial density fluctuations that gave rise to today's Universe.
But only in regions where inflation has ended, and where this energy inherent in space is transformed into matter and radiation, and the Big Bang happens.

And in these regions - where inflation has ended - we have a Universe, much larger than its observed part. This is the idea of the multiverse, and that is why we believe that we most likely live in it.

What about this inflation itself? Can we find out anything about her?
You can decide that quantum fluctuations - and the density fluctuations they sowed - are all that we have. And until recently, I would have told you so. But in theory, inflation also generates gravitational waves, which we still could not detect. LISA, a space-borne laser interferometer antenna (project, relegated at best in the 2030s), was our best hope for direct wave detection.

But even without LISA, gravitational waves can be detected by an indirect method. Although gravitational waves and light travel at the same speed, light slows down as it passes through the medium. This happens even in such a rarefied medium as intergalactic and interstellar space! And since gravitational waves do not slow down - only the curvature of space-time affects them - they overtake the light and themselves lead to polarization!

In general, it is precisely the deformations of space-time on certain scales that stretch the waves of light in a certain way when they travel from the Big Bang to our eyes.

Specifically, the characteristic features of gravitational waves should appear as B-mode polarization, and they should leave a specific pattern on a large scale.
Although the Plank Observatory should see and confirm this, it was ahead of the team at the South Pole: BICEP2!

On a scale of about 1.5 degrees, the B-mode of polarization is very obvious, and it has already been declared open, albeit with a 2.7σ significance (note: on these scales, the significance is 5.2σ, but they need to convince everyone that this detection level has not appeared thanks to a combination of foreground and systematics). 2.7σ means that there is a 2% chance that this detection is false, and will disappear with the receipt of more data. But in the world of science, this is quite a high probability, therefore, so far this discovery should not be considered a fait accompli.

If the discovery passes the test, it will be a very serious event. That is what we need to measure, and not only to find out whether there was inflation (most likely it was), but to find out which inflation model describes the Universe?
“Plank”, having released the first results last year, did not find anything at all.

There are several general types of inflation that could occur: in particular, if the value of r in these graphs turns out to be zero, this will be in favor of the “small fields” model, and if it turns out to be something huge (for example, 0.2, judging by these results), it will be proof of the “large field” model.

Is this a clear result? Not. We need much better statistics to declare this a discovery - we cannot accept these results and declare: “yes, these are initial gravitational waves remaining from time to inflation,” since we need better proof. 2.7σ is not bad, but in the tough world of physics we need a confirmed result of 5σ. The dustbin of physics history is full of “discoveries” from 3σ, which disappeared with the arrival of new data.
We know that inflation was; the origins of the structure in the universe - its current appearance, its appearance 13.8 billion years ago, and anywhere in between - has already told us about it. But there is an opportunity, and the first hints that gravitational waves could also remain. And if it turns out that we really saw them, we will have to get confirmation of this in the next few years. But if the observation goes insignificant as data is collected, it will not mean that the inflation model is wrong — only that it does not produce the strongest B-modes.

This is not yet a “discovery”, but a hint that we could stumble upon something amazing: the first hint at how our Universe was born. If it turns out to be true, it will be the discovery of the century. But if the new data disproves it - which may well happen - this does not mean that the inflation model is wrong; This means that gravitational waves from inflation are less than predicted by the most optimistic models.
But whether it is real or not, we will still learn a little more about how our entire Universe appeared.
Update: in the comments to the original article, readers reported that the paper mentions significance greater than 5σ. In particular, they look at a certain part of the angular scale, where they actually see a signal with a significance of 5.2σ.

Can focusing be responsible for this? This is the only component that can be deleted - if I, of course, correctly understood the work - with a significance of only 2.7σ.
See for yourself.

The significance of the result is not higher than that of the most probable source of uncertainty, and even if r can be equal to zero, it is very important to exclude this possibility. In her work, they might have been expelled, but it did not seem to me that this was done clearly and clearly. Nevertheless, it is very interesting to me how this will develop! If they exclude focusing in the same way as synchrotron emission, the 5σ limit will be satisfied, and this will already mean the Nobel Prize!
A later note to the article written on March 18, 2014:
On March 17, 2014, scientists from the Harvard-Smithsonian Center for Astrophysics announced the discovery of a B-mode at the level of r = 0.2. However, a later analysis (published September 19, 2014), carried out by another group of researchers using data from the Planck Observatory, showed that the result of BICEP2 can be completely attributed to galactic dust.