Probably there is not a single field of human activity, so full of disappointments and rejected heroes, as attempts to create thermonuclear energy. Hundreds of reactor concepts, dozens of teams that consistently became favorites of the public and state budgets, and finally sort of decided to be the winner in the form of tokamaks. And here again - the achievements of Novosibirsk scientists revive interest around the world in the concept cruelly trampled in the 80s. And now more.
GDL open trap with impressive resultsAmong the variety of proposals, how to extract energy from a thermonuclear fusion is most of all oriented towards the stationary confinement of a relatively loose thermonuclear plasma. For example, the ITER project and the wider - toroidal tokamak traps and stellarators are from here. They are toroidal because this is the simplest form of a closed vessel that does not work out of magnetic fields (because
of the hedgehog combing theorem, a spherical vessel cannot be made). However, at the dawn of research in the field of controlled thermonuclear fusion, the favorites were not traps of complex three-dimensional geometry, but attempts to keep the plasma in the so-called open traps. These are usually also magnetic vessels of cylindrical shape in which the plasma is well kept in the radial direction and leaks from both ends. The idea of ​​the inventors is simple here - if the thermonuclear reaction of the plasma will go faster than the heat from the leaking from the ends, then God bless him, with the openness of our vessel, the energy will be generated, and the leak will still occur in a vacuum vessel and the fuel will be walk in the reactor until it burns down.
The idea of ​​an open trap is a magnetic cylinder with plugs / mirrors at the ends and expanders behind them.')
In addition, in all open traps, various methods are used to keep the plasma from escaping through the ends - and the simplest one here is to sharply increase the magnetic field at the ends (put magnetic “plugs” in domestic terminology or “mirrors” in the west), while the incident charged particles will, in fact, spring off from the mirror mirrors and only a small part of the plasma will pass through them and fall into special expanders.
And a little less schematic representation of the heroine of today - a vacuum chamber is added, in which plasma flies, and all equipment.The first experiment with a “mirror” or “open” trap - Q-cucumber was made in 1955 in the American
Lawrence Livermore National Laboratory . For many years, this laboratory has become a leader in the development of the TCF concept based on open traps (OL).
The world's first experiment - an open trap with magnetic mirrors Q-cucumberCompared to closed competitors, the advantages of the OL can be written in a much simpler geometry of the reactor and its magnetic system, which means low cost. So, after the fall of the first leader of the UTS - Z-pinch reactors, open traps receive maximum priority and funding in the early 60s, as promising a quick solution for little money.
Early 60s, Table Top TrapHowever, the same Z-pinch did not retire accidentally. His funeral was associated with the manifestation of the nature of the plasma - instabilities that destroyed plasma formations when trying to compress the plasma with a magnetic field. And it is this poorly studied feature 50 years ago that immediately began annoyingly disturbing experimenters with open traps. Grooved instabilities cause the magnetic system to become complicated by introducing, apart from simple round solenoids, the “Ioffe stick”, “baseball traps” and “yin-yang coils” and reduce the ratio of the magnetic field pressure to the plasma pressure (parameter β).
"Baseball" superconducting magnet trap Baseball II, mid 70sIn addition, plasma leakage is different for particles with different energies, which leads to plasma non-equilibrium (i.e. non-Maxwell velocity spectrum of particles), which causes a number of unpleasant instabilities. These instabilities, in turn, “swinging” the plasma accelerate its escape through the end mirror cells. At the end of the 60s, simple variants of open traps reached the limit in temperature and density of the confined plasma, and these figures were much smaller than those needed for a thermonuclear reaction. The problem was mainly in the rapid longitudinal cooling of electrons, which then lost energy and ions. Needed new ideas.
Successful TMX-U Ambipolar TrapPhysicists offer new solutions related primarily to improving the longitudinal plasma confinement: ambipolar confinement, corrugated traps, and gas-dynamic traps.
- Ambipolar confinement is based on the fact that electrons “flow out” from an open trap 28 times faster than deuterium and tritium ions, and a potential difference occurs at the ends of the trap — positive from the ions inside and negative from the outside. If at the ends of the installation we make field amplifications with dense plasma, then the ambipolar potential in a dense plasma will keep the internal less dense contents from expansion.
- The corrugated traps create a “ribbed” magnetic field at the end, in which the heavy ions scatter due to the “friction” of traps trapped in the “hollows” of the field.
- Finally, gas-dynamic traps create by magnetic field an analogue of a vessel with a small hole, from which the plasma flows at a slower rate than in the case of “mirror-plugs”.
Interestingly, all these concepts, according to which experimental facilities were built, required further complication of the engineering of open traps. First of all, here, for the first time, complex accelerators of neutral beams appear in the TCB, which heat the plasma (in the first installations, heating was achieved by an ordinary electric discharge) and modulate its density in the installation. Radio frequency heating is also added, which first appeared at the turn of the 60s / 70s in tokamaks. Large and expensive Gamma-10 plants are being built in Japan, TMX in the USA, AMBAL-M, GOL and GDL in the Novosibirsk INP.
The scheme of the magnetic system and plasma heating Gamma-10 well illustrates how far from simple solutions of the OL to the 80th years.In parallel, in 1975, on a 2X-IIB trap, American researchers were the first in the world to reach a symbolic ion temperature of 10 keV, which is optimal for the flow of deuterium and tritium thermonuclear burning. It should be noted that in the 60s and 70s they passed under the sign of chasing the right temperature at least in any way, since The temperature determines whether the reactor will work at all, while the other two parameters — the density and rate of energy leakage from the plasma (or more commonly called “holding time”) can be compensated for by increasing the size of the reactor. However, despite the symbolic achievement, 2X-IIB was very far from what would be called a reactor - the theoretical power output would be 0.1% of the plasma spent on retention and heating. The low temperature of electrons remained a serious problem — about 90 eV against a background of 10 keV ions, due to the fact that electrons were somehow cooled on the walls of the vacuum chamber in which the trap was located.
Elements of the now not operating ambipolar trap AMBAL-MIn the early 80s the peak of the development of this branch of the TCB. The peak of development is the American project MFTF worth $ 372 million (or 820 million at current prices, which brings the project closer to the cost of a car like the Wendelstein 7-X or the K-STAR tokamaku).
MFTF Superconducting Magnetic Modules ...

And the body of its 400 ton superconducting magnet endIt was an ambipolar trap with superconducting magnets, incl. mastery end "yin-yang", numerous systems and heating plasma diagnostics, a record in all respects. It was planned to achieve Q = 0.5, i.e. the power output of thermonuclear fusion is only two times less than the cost of maintaining the operation of the reactor. What are the results achieved by this program? It was closed by a political decision in a state close to launch readiness.
Trailer "Yin-Yang" MFTF during installation in a 10-meter vacuum chamber installation. Its length was to reach 60 meters.Despite the fact that this is a shocking decision on all sides, it is very difficult to explain, I will try.
By 1986, when MFTF was ready to launch concepts in the sky, TCB had a star of another favorite. A simple and cheap alternative to open-air traps, which by this time had become too complicated and expensive against the background of the initial concept of the early 60s. All these superconducting magnets of puzzling configurations, fast neutral injectors, powerful radio frequency plasma heating systems, puzzling schemes for suppressing instability such complex installations will never become a prototype of a thermonuclear power station.
JET in the original limiter configuration and copper coils.So tokamaks. In the early 80s, these machines reached plasma parameters sufficient for burning a thermonuclear reaction. In 1984, the European JET tokamak was launched, which should show Q = 1, and it uses simple copper magnets, its cost is only $ 180 million. In the USSR and France, superconducting tokamaks are being designed, which almost do not spend energy on the operation of the magnetic system. At the same time, physicists who have been working on open traps for years cannot make progress in improving plasma stability, electron temperature, and promises on the achievements of the MFTF are becoming increasingly vague. The following decades, by the way, will show that the bet on tokamaks turned out to be relatively justified - it was these traps that reached the level of power and Q, which are interesting to the power engineers.
The success of open traps and tokamaks to the beginning of the 80s on the map of the "triple parameter". JET will reach a point slightly higher than TFTR 1983 in 1997.The MFTF decision finally undermines the position of this direction. Although the experiments at the Novosibirsk Institute of Nuclear Physics and the Gamma-10 Japanese installation are continuing, quite successful programs of the TMX and 2X-IIB predecessors are being closed in the United States.
The end of the story? Not. Literally before our eyes, in 2015, an amazing quiet revolution is taking place. Researchers from the Institute of Nuclear Physics. Budker in Novosibirsk, which consistently improved the GDL trap (by the way, it should be noted that ambipolar rather than gas-dynamic traps excelled in the west) suddenly reached plasma parameters, which were predicted as “impossible” by skeptics in the 80s.
Once again GDL. Green cylinders protruding in different directions are neutral injectors, which are discussed below.The three main problems that have buried open traps are the MHD stability in an axisymmetric configuration (which required complex-shaped magnets), the imbalance of the ion distribution function (microinstability), and the low electron temperature. In 2015, the GDL, with a beta value of 0.6, reached an electron temperature of 1 keV. How did this happen?
Avoiding axial (cylindrical) symmetry in the 60s in an attempt to defeat the flute and other plasma MHD instabilities, in addition to complicating magnetic systems, also led to an increase in heat loss from the plasma in the radial direction. A group of scientists who worked with the GDL used the idea of ​​the 80s to apply a radial electric field creating a vorticized plasma. This approach led to a brilliant victory - at beta 0.6 (recall that this ratio of plasma pressure to magnetic field pressure is a very important parameter in the design of any fusion reactor - since the rate and density of energy release is determined by plasma pressure, power of its magnets), compared to the tokamachny 0.05-0.1 plasma is stable.
New measuring instruments, "diagnostics", allow a better understanding of plasma physics in the GDLThe second problem with microresistance caused by the lack of ions with low temperatures (which are pulled from the ends of the trap by ambipolar potential) was solved by tilting the injectors of neutral rays at an angle. This arrangement creates, along the plasma trap, ion density peaks that keep “warm” ions from escaping. A relatively simple solution leads to the complete suppression of microinstability and to a significant improvement in plasma confinement parameters.
The neutron flux from thermonuclear burning of deuterium in the GDL trap. Black dots - measurements, lines - different calculated values ​​for different levels of microinstability. Red line - micronestability suppressed.Finally, the main “gravedigger” is the low electron temperature. Although thermonuclear parameters are reached for ions in traps, high electron temperature is the key to keeping hot ions from cooling, and therefore to high Q values. The low temperature is caused by high thermal conductivity “along” and the ambipolar potential sucking the “cold” electrons from the expanders behind the ends traps inside the magnetic system. Until 2014, the electron temperature in open traps did not exceed 300 eV, and in the GDL, psychologically important 1 keV was obtained. It was obtained due to the fine work with physics of the interaction of electrons in terminal expanders with a neutral gas and plasma absorbers.
This turns the situation on its head. Now the simple traps threaten again the superiority of tokamaks that have reached monstrous size and complexity (
some examples of the complexity of the ITER
systems ). And this is the opinion of not only scientists from the INP, but also of serious
American scientists , published in reputable journals.
Another GDL near. For photos thanks dedmaxopkaSo far, however, the success of the GDL has led to
new proposals for installations only at INP itself. Having won a grant from the Ministry of Education and Science of 650 million rubles, the institute will build several engineering stands, within the framework of the promising rector "
GDML-U ", combining the ideas and achievements of the GDL and a way to improve the longitudinal retention of the GOL. Although under the influence of new results, the image of GDML is changing, but it remains the main idea in the field of open traps.
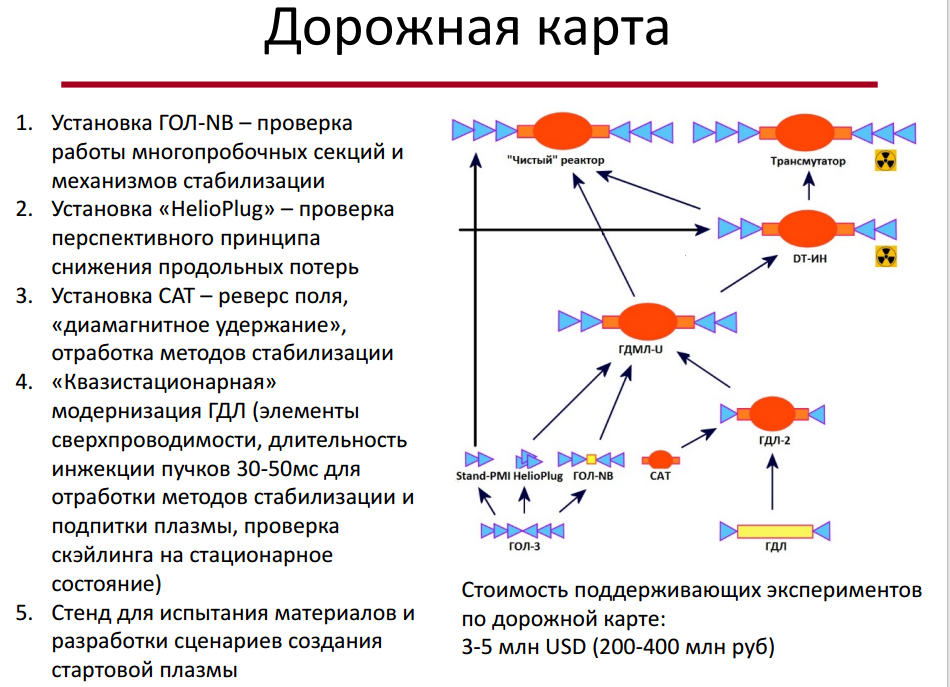
Where are current and future developments compared to competitors? The tokamaks, as you know, have reached Q = 1, have solved many engineering problems, will send to the construction of nuclear rather than electrical installations and are confidently moving towards the rather typical of a power reactor with Q = 10 and fusion power up to 700 MW (ITER). Stellarators who are a few steps behind are moving away from studying fundamental physics and solving engineering problems with Q = 0.1, but for now do not risk entering the field of truly nuclear facilities with thermonuclear tritium burning. GDML-U could be similar to the
W-7X stellarator in terms of plasma parameters (being, however, a pulsed installation with a discharge duration of a few seconds versus a half-hour W-7X in perspective), however, due to simple geometry, its cost can be several times less German stellarator.
Evaluation of the INP.There are options for using GDML as a facility for studying the interaction of plasma and materials (there are quite a few such plants in the world, however) and as a thermonuclear source of neutrons for various purposes.
Extrapolation of the size of GDML depending on the desired Q and possible applications.If tomorrow open traps will again become favorites in the race to the TCF, one would expect that due to smaller capex in each stage, by 2050 they will catch up and overtake tokamaks, becoming the heart of the first thermonuclear power plants. Unless the plasma gives new unpleasant surprises ...