Hello! Together with Dmitry Zimin's Book Projects, we released a book by Jamie Davis:
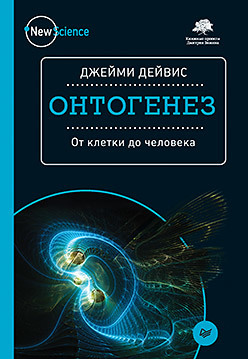
How have we become what we are? Why do we have two arms and legs, but only one head? Why is the human body symmetrical, but at the same time its halves are not completely identical? Why are the fingerprints of identical twins not the same? How did our brain develop and what is consciousness? Why are we mortal and what is the biological meaning of it?
Similar questions people have asked themselves since ancient times. Even now, with the modern development of science, the fundamental principles are not fully understood, thanks to which a single fertilized egg forms such a complicatedly organized organism consisting of a multitude of molecular structures that interact with each other, have their own life cycle, are capable of regeneration and self-development. “At the core of this is the principle of central adaptive self-organization,” says modern biology. But what is this principle?
')
Jamie Davis did a great job of adapting the most complex scientific material to a level understandable to the general reader. In a fascinating and ironic form, having supplied his story with more than 80 illustrations, the author invites the reader to travel through all aspects of human biological development, from conception to death. Recent advances in embryology, genetics, physics, neuropsychology will allow us to learn more about stem cells and protein metabolism, the differences between chromosomes and functions of genes, neural connections and other major factors affecting the internal evolution of man.
Ethical statement
This book describes the mechanisms of human development. It contains published in scientific publications information obtained in the study of human embryos, as well as during animal experiments. Since academic publishers and research foundations require that work be verified by the relevant independent ethics commissions, I made the assumption that the experiments cited in this book met the standards of the time when they were conducted. Ethical standards are constantly changing, and some studies that were conducted many years ago are now banned. Mentioning the results of specific experiments in this book does not imply personal ethical approval of the methods used in them by either the author or the publisher of the book.
Introduction
Chapter 1.
Encounter with technology alien to us
In these words, the English philosopher and poet Samuel Coleridge expressed in poetic language the surprise of a child who first asked his parents: “How did I come into the world?” Many adults believe that this question concerns sexual relations and begin to painfully think about what can be said and when . However, the child does not care about these psychosocial difficulties, his question is even simpler and deeper - how can a new person appear?
No child has yet received a full and correct answer, because none of the adults knows for sure. In Coleridge's time, separate facts were known about the sequence of anatomical changes occurring as the new man grows in the womb, but how and why they occur remains a mystery. Two subsequent centuries, scientists have tried to understand how the fertilized egg turns into a child. Over the past decade, science has made great strides forward, but as scientists decipher the most complex mechanisms and solve concrete riddles, the general sense of amazement only grows. The history of embryonic development, which has so far been discussed mainly in specialized scientific articles, is truly amazing. This story is about what happened to each of us, and therefore should be in the public domain. I was lucky to work in this area, and in this book I tried to collect the most significant results of modern research and answer the most profound — and most childish — question: how did I come into the world?
Our understanding of human embryonic development was not formed within a single scientific approach, but was the result of the generalization of a huge amount of information from different fields of knowledge. Embryology and neonatology, directly studying the development of man, have put at our disposal a large amount of anatomical and functional information. Genetics and toxicology, areas broader than developmental biology, are of great importance for identifying the causes of congenital anomalies. This is very important because, knowing these reasons, it is possible to identify cascades of biochemical reactions (the so-called molecular paths) necessary for the normal development of the relevant parts of the body. Biochemistry and molecular biology are indispensable for identifying the details of the work of the molecular pathways involved in development, down to the level of interaction of atoms of biological molecules. Cell biology makes it possible to explain how, through the interaction of different molecular paths, control of the behavior of individual cells is provided. Exploring a higher level of organization, physiology, immunology, and neuroscience reveal ways to communicate and coordinate a multitude of cells.
All the disciplines mentioned are in the fields of medicine or biology where research on human embryology has traditionally been carried out. However, recently the fields of science that at first glance have no relation at all to this topic: mathematics, computer science, and even philosophy have contributed to the understanding of human development. They did not clarify specific details (what and when does a particular cell do), but touched on fundamental developmental issues, for example: how can a simple thing become complicated? How can development mechanisms unsustainable with respect to random errors provide high accuracy of reproduction of the final result? and is it too difficult for a person to develop so that even intellectually developed people can fully understand him? The last of these questions remains open, and the subject of the dispute is the word "completely." However, significant progress was achieved in resolving the first two questions. The answer lies in two related concepts: "emergence" and "adaptive self-organization." These are actually two sides of the same phenomenon. "Emergence" is the emergence of complex structures and behaviors from simple components and rules; This term is usually used by those who look at the system “down” from the position of “high-level behavior”. “Adaptive self-organization” is a “look up”; This term allows us to describe how the application of these simple rules to the components of a system leads to their collective behavior — the fulfillment of complex and delicate tasks of large spatial scale.
It is thanks to adaptive self-organization that non-living molecules can create a living cell, and cells with limited individual abilities can form a multi-cellular organism capable of much. Adaptive self-organization is the leitmotif of my book, as it lies at the heart of developmental biology. The concepts of “adaptive self-organization” and “emergence” are beyond the scope of biology, and in the “Additional literature” section I cited several references to fascinating books on this topic.
New data on developmental biology makes it clear that the organism does not arise at all in the way buildings or machines are built. It's funny, but true: the ways of forming our own body are completely alien to our ideas about how it could be. Therefore, trying to understand how the embryo builds itself, it is very useful to compare - and contrast - the development of this biological system with the usual methods of building objects.
All engineering projects, whether locomotive assembly or building construction, have common features. First of all, any project has a specific plan - it can be a drawing or some other scheme - clearly showing what we want to get in the end. The plan shows the expected result, but it will not be part of this result. Each project has a leader — the chief engineer or architect — who gives instructions to subordinates, and those in turn to the workers who perform the laying of bricks, cutting, welding and painting. Details of the future design cannot connect together on their own. This is done by workers — bricklayers, assemblers, welders — who themselves are not part of this design. At the same time, workers and the chief engineer own a huge amount of “external” information — on welding technology or stone-making business — which is not present in the objects they create. And finally, most of the man-made structures are put into operation only after the completion of work.
In biological design, we will not find these familiar steps. This once again emphasizes the difference between living beings and engineering structures. Unlike technical projects, biological design does not imply any drawings and sketches of the final result. Of course, the fertilized egg contains information (in the genes, in molecular structures, in the spatial distribution of the concentrations of chemical substances), but the relationship between this information and how the finished organism will ultimately look is far from simple. It is known that this information controls the further sequence of events (and we know this, because changing this information, for example, when a gene mutates or changes in the concentration of a certain substance in a certain place, changes the sequence of events, and development proceeds in an abnormal way).
In technology, and especially in mathematics, the final result can be achieved with step-by-step instructions. Consider an example: in the middle of a wheat field, insert a stake into the ground and tie a rope to it. Take the other end and walk a few meters to make the rope stretch. Then go right, keeping the tension. This way you can draw a simple circle. Some structures are much easier to create by instructions than by drawings. If you have a pencil and paper at hand, try using the instructions below to draw a geometric figure called “Sierpinski Napkin”.
1. Draw an equilateral triangle with a horizontal base. The more it is, the better. We will consider it a “source triangle”.
2. Inside this triangle draw three segments. Each of them must pass from the middle of each side into the middle of the adjacent. These segments form an inverted triangle, which occupies a quarter of the original area.
3. Hatch the resulting triangle.
4. Now you see three open triangles inside the original. Perform the same operations on each of them as on the original triangles, starting from point 2.
5. (Continue until you get bored: if you are armed with a good pencil, this activity can last forever.)
“Sierpinski Napkin” (thanks to the shaded areas, the drawing resembles openwork knitting) is an example of a fractal structure. At any magnification we get the same image. Another example of a fractal is the “Cantor set”. It is most convenient to paint on the surface, which is easy to erase. School board will do. Draw a line, then erase its middle third, then erase the middle thirds of the two lines obtained, and so on. After some time you will get a lot of points located at certain intervals. The statistical properties of these intervals are identical to the properties of many natural phenomena, be it the shedding of sand from a sand dune or the intervals between drops of water from a leaking tap, earthquakes, epidemics, and cases of mass extinction of animals.
Step-by-step instructions, not sketches, are used to create objects not only in mathematics, but also in everyday life; The simplest example is the recipe. The same principle works and textile production, from hand knitting ("knitting one loop, throwing one") to "Jacquard machine" (1801), the world's first industrial robot, on which it was possible, by changing punched cards, to switch difficulty levels from the simplest to the most complex pattern. The music is also reproduced thanks to the instructions, the role of which is played by the note signs on the stave, by which the musician can play sounds of the required pitch and duration at the right time.
The centuries-old experience of using instructions to obtain a conceived result with minimal time and effort leads to the fact that we tend to think that biological information determines our appearance in some similar way. This is a dangerous delusion. There is a significant difference between living organisms and man-made objects: in the latter case, the instructions are followed by an external conscious agent of action. Even seemingly obvious exceptions, such as an automatic knitting machine or a mechanical piano, were created according to instructions and plans by the same external agents, which means that they are not exceptions. Simply put, cardigans, symphonies, cars and cathedrals did not create themselves. Following the instructions, introducing the necessary information about the process (the ability to knit, prepare or lay a brick) and the actual work with materials are carried out not by the growing structure, but from the outside. On the contrary, the information contained in the embryo is read and processed by the embryo itself; he has no one to pass on either hard physical work or thinking about optimizing the process. As we shall soon see, this means that the responsibility for biological design lies with all its participants, and not with the manager, as in the case of engineering projects. The process of creating a human body is not controlled by individual parts of the embryo, but by the system as a whole.
To understand the features of the construction process, it is also necessary to have some idea of ​​the nature of the materials used. Next to my lab at Edinburgh University are three famous bridges: the elegant Dean Bridge, built by Thomas Telford, the legendary Bay Bridge, built by Benjamin Baker, and, not far from it, the Ford Road Road Bridge. Telford built a bridge of stone blocks - heavy, bulky, reliable only due to compressive stress. Therefore, he used the traditional method: first, supports were built, then a wooden frame was constructed for the arched span, then stones laid out in the form of an arch were laid on it. After the weight of the stone stabilizes the span, the frame can be removed.
Baker used for the construction of the railway bridge a radically new material for those times - steel. This material can be held both by stretching and compressive stress, so the construction could be started from any support by attaching sections to it with one end. Cranes were used to place the long and relatively light steel sections in place. Between themselves, these sections are connected with rivets.
The cable-stayed bridge, the newest of the three, is held by steel cables, cables, which are fixed on pylons on different banks. In this case, the pylons were first installed, then the support points for fixing the cables were planned, and then the bridge-holding guys were gradually tightened.
In each of these cases, the bridge construction strategy was determined by the nature of the materials. None of them could be built using a strategy designed for a bridge of a different type. Similarly in biology: the design strategy depends on the nature of the components involved. Thus, it is time to introduce you to the three key biological components that will be mentioned many times in this book - proteins, messenger RNA (mRNA) and DNA.
Proteins are the main building materials in biology. Of these, most of the physical structures that give shape to the cells are created; they form channels and pumps that regulate the circulation of substances in the cells. In addition, proteins - catalysts. They trigger and control biochemical reactions and metabolic pathways, the products of which are other components of the body, such as DNA, fats and carbohydrates. The relative importance of proteins can be illustrated, for example, by the following fact: erythrocytes (red blood cells) lose nuclei in the process of maturation, which contain all their genes, but after that they still live for about a hundred and twenty days. The cell in which the genes are preserved, but the function of proteins is violated, will die within a few seconds.
Protein consists of a long chain of individual blocks - amino acids. There are about twenty types of amino acids that differ in structure and chemical properties. They interact with each other, and this means that chains of amino acids can twist into intricate shapes - spontaneously or under the action of other proteins. This process of twisting is so complex that it is impossible, knowing only one sequence of amino acids, to predict which protein will be obtained as a result. (Computer programs for predicting the shape of a protein exist, but they use a combination of calculations and probabilistic reasoning based on the already known structure of proteins and amino acid sequences, which were experimentally detected using X-ray crystallography. Thus, these programs are similar to computer programs that are used by weather forecasters; however, it should be noted that the prediction of the protein structure is still more accurate than the weather forecast.)
Different proteins are made up of different amino acid sequences. One after another, they join the growing chain of the protein in the order that is established by a molecule called messenger RNA (abbreviated mRNA) (Fig. 1). An mRNA molecule is also a single chain of individual blocks — nitrogenous bases: adenine (A), cytosine ©, guanine (G), and uracil (U). They are similar in structure and compared to amino acids are not so interesting in terms of chemical properties: mRNA molecules do not play a large role in the cell besides the regulation of the sequence of amino acids in the forming protein. This sequence is determined by the sequence of bases in the mRNA. Each amino acid has its own code of three nitrogenous bases.
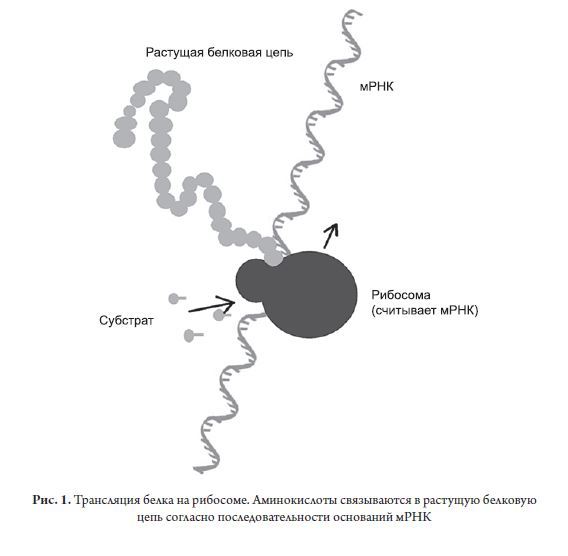
The sequence of bases in mRNA molecules is determined by the sequence of bases in DNA. DNA is a very long molecule consisting of combinations of four nitrogenous bases: adenine, cytosine, guanine, and thymine (T), which can be arranged in different sequences. Separate DNA molecules that make up most of the forty-six chromosomes in each cell of our body contain millions of nitrogenous bases. Some parts of this chain are genes. When genetic information is read, the RNA molecule encodes the DNA base sequence (A, C, G, T) in the language of its bases (A, C, G, U). Thus, RNA is essentially a copy (transcript) of a gene in a different medium. The actual reading of the genes is made by whole protein complexes. First, they bind to different short base sequences at the beginning of the gene, ATAAT or TCACGCTGA. Different genes have different combinations of such short sequences that mark their beginning, and each sequence is associated with a specific protein. Thus, different combinations of proteins are involved in the activation of the reading process of various genes.
The fact that different genes are activated by different DNA-binding proteins is very important, because different cells of the body must synthesize different types of proteins. For example, intestinal cells produce proteins that allow food to digest, ovarian cells synthesize proteins for sex hormones, and white blood cells produce proteins to fight microbes. All of these cells contain all the genes of the genome, even those that they will never need. However, only the genes necessary for specific cells are read, and this happens due to the presence of "exclusive" DNA-binding proteins.Now, willy-nilly, we will have to abandon the idea that any of these components may be responsible for the development of the cell - or the embryo - as a whole. I repeat: proteins are formed only because their formation is dictated (by means of mRNA) active genes. In turn, these genes are active only because they have been activated by already existing proteins. Thus, a vicious circle is obtained: control is not concentrated at any particular point, because it is carried out everywhere (Fig. 2).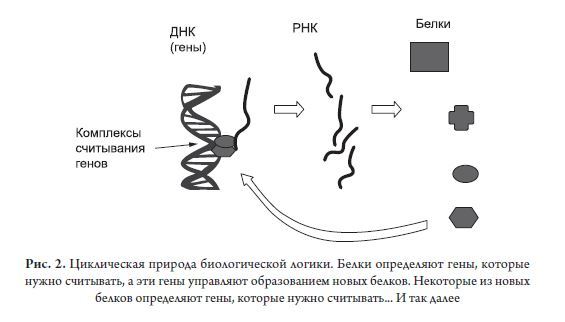
The cycle, whose scheme is shown in Fig. 2, leads to one interesting thought. In order for the cell to remain stable, among the active genes there must be such genes that determine which proteins will bind to the sequences that mark these same genes. At the same time, however, the set of active genes should not include any proteins that activate the currently inactive genes. If these conditions are not met, proteins created by a set of active genes will not be able to maintain the activity of the same set of genes — some of them will “turn off”, others will “turn on”, and as a result a completely different set of proteins will be made, and so on. These changes will continue until a stable state is reached. It is this pattern that underliesas cells of our body are transformed in the process of development into cells of new types. Such a change, as a rule, occurs under the influence of external signals, which change the ability of specific proteins to activate genes: they break stability and cause a transition to a new state. We will constantly encounter examples of such signals in subsequent chapters of the book."Cyclic" control, distributed throughout the system, is by no means the only strange feature of biological design. There is another feature that seems fantastic if we consider it from the standpoint of traditional engineering. Its essence is that biological molecules can spontaneously unite into structures of a larger spatial scale. Bricks and bolts are definitely not capable of this! This process, which is crucial for life, is a bit like growing crystals. Ordinary crystals, as in the sets of a young chemist, are formed because their constituent molecules can bind with each other, as a rule, due to local electric charges. Proteins also have local electric charges, often located in hard-to-reach areas inside the protein or on its convex parts.The charge distribution and the shape of the protein is dictated by the amino acid sequence. Sometimes a protein molecule is concave in front and convex in the back, so that the convexity of one protein coincides with the concave part of the other, like the details of the Lego designer. In this case, protein molecules can line up “head to tail” in a thin structure of arbitrarily large length (Fig. 3). More often, however, a protein can recognize binding sites not only in other proteins or any other molecules, but also in its own structure. This means that it cannot create endless threads with identical molecules, as it happens in crystals, but binds only with a certain number of proteins, forming multicomponent complexes of a particular structure. These complexes play an important role in cells, because they act like tiny machines,which can carry out complex chemical reactions or even organize the assembly of structures that are too large and complex for spontaneous organization. An example is the above-mentioned protein complexes for reading genes.
The level of organization of protein complexes brings us to a very important point. The organization of proteins into complexes is based on information that is found only in the proteins themselves (“information” is in this case a synonym for structure). This is essentially a chemical process, and its result is always the same - it is reliable, reproducible, but unchanged. At higher levels of organization, biological structures are more variable, they adapt to certain conditions. For example, the shape of a cell depends on its place in the composition of the tissue. Its connections with neighboring cells are determined by their mutual arrangement. Such formations cannot be determined solely by the information embedded in the chemical structure of their molecular components; additional information is required. So we move from an internally driven structure to a structure thatregulated, including from the outside, we return from pure chemistry to biology.In biological systems, multi-level regulation is added to chemical self-assembly, and as a result, systems are obtained in which the structures adapt to environmental conditions. At this stage, the concept of adaptive self-organization mentioned above is of particular importance. It turns out to be the key to understanding how just a few thousand genes and proteins, having no idea about the structure and functions of the human body as a whole, can nevertheless build it. What a contrast with engineering projects, in which external agents of action, be they workers or robots, are absolutely necessary for the correct assembly of components! The following chapters will discuss the importance of adaptive self-organization for human development at various levels, from the self-organization of molecules within the cell to the formation of complex tissues.Biological construction has one more unusual feature, and it is connected with the constraint that lies at the very foundation of life: it cannot be stopped to reflect, and then start over. Mechanisms created by humans, such as computers and airplanes, should function only after the completion of work, and while the assembly process is in progress, nothing is required of them. The development of the embryo is accompanied by a strict condition: at all stages of development, it must remain alive. If the plumber wants to put a cut, he blocks the water and installs a T-shaped pipe on the main pipe. When work is completed, water can be turned on again. And if such an approach was used when creating the human body, for example, when a new vessel was being withdrawn from the aorta? The fruit would die right there. The same applies to other important systems of the body.The indisputable requirement of constant maintenance of vitality in the development of the organism is a very serious condition. This is another reason why the development of the human body may seem so strange and so complicated compared to the usual methods of construction.Trying to understand the earliest stages of our existence, we must be ready to reject the usual ideas about the process of creating things and look at the development of the embryo in the light of its own laws. This is a trip to uncharted territory, it requires a new way of thinking. And no engineering metaphors! In the end, we do not create embryos, they create us.»More information about the book can be found on
the publisher's website.» Home» FragmentFor readers of this blog 25% discount coupon - Ontogenesis