The third of a series of articles devoted to the description of the main differences between low-power long-range radio communication technologies, which is now spreading in IoT systems: LoRa broadband from narrowband (UNB, Ultra Narrow Band) systems, such as Sigfox and Strig, as well as their practical application .
Hi GT.
')
After the first two articles, as well as living stories on this topic, I was asked several times to tell more about the basic technical aspects of the work of LoRa and UNB networks in somewhat more detail than I described in the first article:
- Channel separation in UNB systems
- The problem of feedback in UNB-systems
- Channel separation in LoRa
- Adaptive speeds in UNB and LoRa
- Immunity in UNB-systems and in LoRa
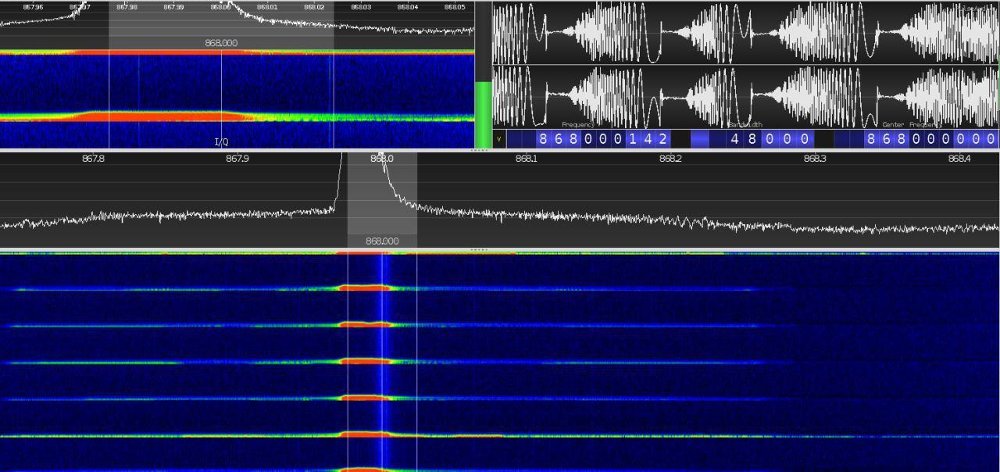
Well, let's get started. Below there will be, as usual, a lot of text and few pictures.
Channel separation in UNB systems
One of the advantages of UNB systems is their effective use of the radio frequency spectrum - in Russia, with minimal restrictions, only a modest bandwidth of 500 kHz (868.7–869.2 MHz) is available, but with a width of the working channel of the UNB system only 100 Hz of such channels even it can fit thousands.
However, frequency division (FDMA) exists only to the extent that there is a carrier frequency — which differs in different channels. In a conventional radio receiving path, after the input amplifier, there is a demodulator whose task is to isolate the low frequency component of the signal, removing the high frequency carrier. Obviously, after such a demodulator, several frequency channels will merge into a single mess, so no FDMA will work.
Even the most difficult problem has a simple and obvious wrong solution. Such a solution in a UNB system would be the use of several radio channels tuned to different frequencies. It would seem - ten channels will allow to receive ten subscribers at the same time, and everyone will be happy. Alas.
One of the serious technical problems of UNB-systems is the frequency instability of quartz resonators, which determine the operating frequency of the subscriber unit. A typical good quartz has a total error of ± 25 ppm, that is, at a carrier frequency of 869 MHz, the actual deviation from it can reach 21.725 kHz. As a result, it is completely meaningless to indicate the exact frequency of the channel on which the subscriber device is broadcasting - in reality it will be plus or minus a bast. There is no way to clarify this frequency in advance - apart from using expensive temperature-compensated oscillators (TCXO) instead of quartz resonators, which can reduce the error by a factor of ten.
Therefore, in a BS with multiple radio channels of reception, these channels will have to be separated from each other by an amount determined by the accuracy of the resonators of the subscriber devices. Technically, this can be done, but a beautiful picture of how effectively UNB uses every available hertz of the spectrum will have to be thinned out a little.
A more advanced version, which is used in practice in serious BSs, is the digitization and subsequent spectral analysis of a wide range of radio (tens to hundreds of kilohertz) in real time. That is, for example, the BS receives a wide band of 100 kHz, digitizes it, finds individual signals of subscriber devices in it, somehow randomly scattered across this band, selects each of them and transmits further to the signal decoders. In fact, a modern base station UNB-system is a very high-performance SDR receiver, paired with a no less productive chip, grinding the data obtained from the receiver.
Such a system really allows you to receive a large number of subscriber devices at the same time, but this is digital signal processing at a frequency close to gigahertz in real time, that is, a hard computational task that requires very serious resources from the BS. The lack of such resources will mean the need to cut either the bandwidth or the maximum data transfer rate.
The practical consequence of this is the difficulty of creating low cost and, at the same time, efficient base stations for UNB systems. BS Sigfox costs around 3,000 euros, the cost of BS Strizha is not reported on the site (but is unlikely to be less than 100 thousand rubles), one of the most popular BS LoRaWAN - Kerlink - costs 1,200 euros. At the same time, Kerlink can be considered an expensive solution, the price tag of which is largely due to not very high natural demand for base stations. A ready-made board with a SX1301 transceiver, to which for getting a full-fledged BS it remains to add a little brain, an interface for uplink, a case and power, costs $ 180. In general, as the production of LoRa increases, there is much to fall in price - despite the fact that even now Sigfox looks pale against its background.
In addition, it also follows from this that when you are offered some kind of cheap femtocell for a UNB system, you need to carefully study what is inside of it and what are its real performance characteristics.
The problem of feedback in UNB-systems
The large relative instability of the frequency of the frequency-generating quartz resonator is the direct cause of one of the most significant - from the point of view of practical application - problems of UNB-systems: the technical impossibility of symmetric feedback, that is, the BS → subscriber communication.
For the subscriber to receive something from the BS, the BS must broadcast on its frequency. However, in the UNB system, we simply do not know in advance the working frequency of the subscriber - there is quartz in it, which gives a frequency spread that is hundreds of times larger than the channel width. We simply cannot increase the width of the reception channel at the subscriber either: it will lead to a deterioration of the signal-to-noise ratio, that is, to a decrease in the communication range (we collect noise power from the entire wide channel). It is impossible to implement spectral analysis on the subscriber device - all the more, the computational power is too small.
The only way to transmit something to the subscriber in the UNB system is to wait until he himself goes on the air, determine his actual working frequency on the BS and respond to it on the same.
LoRa has three classes of subscriber devices:
- Class A: having transmitted something on the air, the device waits for a short time to answer from the BS, after which it turns off the receiver until the next communication session.
- Class B: the device turns on the receiver according to a predetermined schedule. The BS knows this schedule and can transfer data to the device in accordance with it.
- Class C: the receiver is always on, the BS can transmit data at any time.
These classes are the result of a tradeoff between power consumption and feedback delay. For example, for many data acquisition devices, feedback, if implemented at all, then in class A: sent data to the BS, received confirmation of its receipt from it.
Another opposite is the outdoor lighting control system (ASUNO), and indeed any control system in general. They need to send a command from the BS to the subscriber at any time, so they use class C devices that continuously listen to the broadcast.
However, here we return to the UNB-systems: since, as mentioned above, the BS can not transmit anything to the subscriber until he himself went on the air, only class A devices are technically possible for them. This is the reason that on Sigfox and Various systems of data collection are being built by Swift, but if you try to find ASUNO using UNB radio, you will fail.
In LoRa, there is no such problem: the bandwidth is such that the system without serious problems is experiencing a subscriber unit frequency drift of two to three dozen kilohertz aside, so feedback works for all classes of devices without any tricks.
Feedback and real network capacity
One of the important points that must be understood in relation to any communication system in IoT, whether it is LoRa or UNB-system - the use of feedback to confirm the delivery of packets from the subscriber to the BS greatly reduces the actual network capacity.
The reason is that, no matter how much the BS of the receive channels, the transmission channel is always the same. Moreover, the transmitter operation excludes any possibility of reception: one antenna is used for transmission and reception, and at the time of transmission the radio path is disconnected from the receiver so as not to kill its input by overloading.
The absence of collisions on the transmission somewhat alleviates the situation: even by accepting data, for example, from half a dozen subscribers, the BS can easily line up the answers to them in a chain so that they do not overlap each other and are transmitted without pauses.
In addition, as a rule, for typical systems, confirmation of data delivery to the subscriber is not required: for example, the meter in the housing and utilities sector can reset the current reading to the BS every day, and if they are not received due to interference on the air today, well, it’s not a big deal. However, if you plan to build a radio frequency network with guaranteed data delivery from subscribers to the BS, you need to take into account that this greatly reduces its capacity.
However, in local networks with not too many subscribers - up to several hundred pieces, for example, for which regular data sending is important, delivery confirmation has the right to life. If the delivery did not take place due to some short-term interference or collision with another subscriber, the end device may try to repeat it several times - until it either receives confirmation from the BS or is convinced that the problem is permanent.
Channel separation in LoRa
Technologically, LoRa is a very interesting invention: if the same Sigfox uses standard modulation mechanisms (differential phase modulation, DBPSK), thanks to which it can work on different chips with the appropriate software stack, then LoRa has developed its own modulation scheme implemented in hardware.
As a matter of fact, LoRa is the radio signal modulation method, although this word is more often called a complex of solutions based on this method.
LoRa is rooted in DSSS — wideband direct-spread-spectrum modulation, in which the original information signal is artificially spread over a wide chunk of the spectrum. The principle of operation of broadband systems directly follows from the Shannon limit (aka Shannon-Hartley theorem) to the maximum speed of error-free data transmission in a channel with a limited bandwidth in the presence of noise:
S = W Ă— log 2 (1 + P / WN 0 ) , where
S - the maximum speed in the communication channel, bps
W - channel width, Hz
P - signal power at the receiver input, W
N 0 - average spectral noise power at the receiver input, W / Hz
P / WN 0 - signal-to-noise ratio (SNR) at the receiver input
On the one hand, the use of a wide band, other things being equal, degrades the signal-to-noise ratio - since the noise is integrated by the receiver from the entire range that it listens to, in the simplest case (if there is no narrow-band interference on the air and N (f) = const), the noise power is directly proportional signal bandwidth. On the other hand, the SNR in the equation is inside the logarithm, but the signal bandwidth is outside, therefore, in practice, an increase in the band turns out to be advantageous from the point of view of increasing the transmission speed.
NB: One of the common misconceptions is the opinion that it is impossible to receive a signal that lies below the noise level. As is evident from the equation, this is not so - it is possible to accept any signal whose power at the input of the transmitter is different from zero, the only question is what data transfer rate you can achieve. All modern IoT-based long-distance communication systems are capable of receiving signals that lie below the noise level.
The developers of LoRa - by the way, in the original it was not Semtech, but a small company, subsequently purchased by Semtech - the task was to adapt DSSS so that it could be effectively implemented in an inexpensive system with low power consumption. Actually, they succeeded. At the same time, unlike UNB-systems, LoRa, as I have repeatedly stressed, is an absolutely symmetrical system, so any chip with LoRa support can act with equal success as both a receiver and a transmitter.
Strictly speaking, the LoRa modulation can be used with very different signal parameters - for example, the SX1276 chips we use in modems support the band from 7.8 kHz to 500 kHz, that is, they can generally work in narrowband mode (albeit at band 62, 5 kHz and less, they will face the same frequency maintenance problem due to the non-ideality of quartz as in UNB systems). However, in practice, as applied to LoRa, one usually speaks of the 125 kHz band and, less often, 250 kHz - because these bands are used in LoRaWAN networks (125 kHz as the main, 250 kHz as an additional high-speed channel). Younger chips such as the SX1272 do not support a bandwidth of less than 125 kHz at all.
The second signal encoding parameter in LoRa is the spreading factor — as the name implies, SF determines how the original signal is spread over a wide spectrum. SF is the primary means of channel separation in LoRa: all LoRa transceivers support 7 SF values ​​orthogonal to each other; that is, in human terms, allowing to distinguish a program encoded with a specific SF, if there are simultaneous broadcasts on the air, encoded with different SF values. In fact, SF is the implementation of code division multiplexing (CDMA) at the physical system level.
SF has a second meaning - it determines the data transfer rate. Specifically, with a 125 kHz bandwidth and seven used SFs, it ranges from 300 bps to 5 kbps. What's the point of this? The fact is that CDMA-separation in itself is not very efficient - besides not too many orthogonal codes, for CDMA to work it is necessary that the simultaneously broadcasting subscribers do not differ too much in power. In the task of extracting the signal of each particular subscriber, all the others who are broadcasting at the moment are noise, which prevents the solution of the problem; As a result, one powerful subscriber is able to block signals from weaker ones.
However, in the case of LoRa, the base station can — and on LoRaWAN networks does just that — give the command to subscribers with a more powerful signal to go to SF at a higher speed. And this means that they will simply free up the air faster - and this is useful both in terms of avoiding collisions and in terms of power consumption: the most voracious part of modern radio systems is the transmitter itself, devouring several tens of milliamperes, and the faster it turns off, battery is better.
As a result, LoRa is not always correctly compared to UNB-systems by subscriber capacity directly: if you take a set of equidistant devices close to the limiting distance, the UNB-systems will be ahead, but if you arrange subscribers at different distances from the BS, as it actually happens - then LoRa can win due to higher transmission rates of subscribers close to BS.
As for the practical issues of the implementation of CDMA in LoRa, then this requires a few hardware demodulators on the receiving device. For full-fledged base stations, Semtech offers the SX1301 chip - there are 49 LoRa “virtual” demodulators in it at once and one more GFSK to keep you from being bored. However, the SX1301 is quite expensive ($ 80), it requires complex external binding (it does not have an integrated radio path) and is sold only directly by Semtech itself - therefore local projects usually use base stations on one or several SX127x series chips officially intended for subscriber devices. One SX127x has one demodulator, but absolutely nothing prevents to put several such chips in a micro-BS - actually, if you put 8 pieces at once, you will get a femtocell capable of fully working with LoRaWAN (it requires 7 × SF in the 125 kHz band and 1 × SF in the 250 kHz band), although it has a lower sensitivity than the full-fledged BS on the SX1301, but it is much cheaper.
NB: Under 49 virtual demodulators, Semtech has in mind a complex scheme in which there are 9 physical demodulators, while one works on a fixed SF, and the remaining 8 can work each with any SF arriving on the air, and also on its own frequency. It is not always correct to count them 48 demodulators, because, obviously, if all eight of them are all on one frequency, they will be able to rake only seven simultaneous messages (according to the number of SF) - but this is indicated by Semtech.
The beauty of LoRa is precisely in its low cost, simplicity and absolute symmetry of solutions for subscriber devices and base stations. If in UNB-systems on BS we instantly plunge into the issues of using multi-gigahertz ADC and digital radio signal processing in real time, then in LoRa
any subscriber device can be used as a micro-BS when the corresponding firmware is installed on it. If desired, a single-channel LoRa base station can be built in the evening from a ready-made LoRa-modem and Raspberry Pi - which is not the case with UNB-systems. This BS allows you to collect data from hundreds of subscribers over an area of ​​several square kilometers - and this is more than enough, for example, for most of the monitoring tasks in agriculture and at production sites.
In addition, the presence of symmetric feedback in LoRa allows you to implement TDMA if you wish: having received data from the subscriber, you must inform him when exactly the next time he is allowed to get in touch. Such a scheme can be especially effective in local networks of object size, where a single base station keeps a register of existing subscribers and scatters them themselves over a temporary grid so that they do not overlap in the air with each other.
In this case, FDMA in LoRa, as a rule, is not used, although technically nothing prevents to make a base station with 2-3 frequency channels. In this case, each subscriber unit will receive a fixed frequency channel - a circuit with a fast pseudo-random frequency tuning in LoRa is inefficient due to the long preamble in the signal of the subscriber unit. In large BSs on the SX1301, this scheme can be implemented on a single chip — the 8 above mentioned LoRa physical demodulators in it allow you to tune to individual frequencies with a deviation of up to ± 2 MHz from the center; but it must be understood that if one demodulator is tuned to one frequency channel, then code division will not work on this channel: subscriber devices can broadcast with any SF, but at any given time the demodulator can process only one specific SF.
Adaptive speeds in UNB and LoRa
If we are talking about adaptive speed in LoRa, then it is worth shedding light on the question why adaptive speeds are not so popular in UNB-systems. Of course, they - with sufficient BS computing power - have fewer problems with subscriber separation, but on the other hand, adaptive speed is not only more free air, but also lower power consumption on the subscriber side.
Alas, in addition to the modest channel width, in UNB-systems, two circumstances make it difficult to increase the speed in excess of the typical 100 bps.
First, it is the performance of the base station processor. As we saw above, for the BS to work effectively, it must perform a spectral analysis of the received broadband signal in real time, extracting from it the narrowband signals of individual subscribers. The words "in real time" here have a very specific quantitative meaning: the FFT calculation frequency is the sampling frequency of the subscribers' signals. Therefore, any attempt to seriously increase the speed of communication with subscribers in a UNB system requires an equally serious increase in the performance of the digital signal processing system. And despite the fact that we are talking about DSP with an initial frequency of almost gigahertz - there is little pleasant demand in such a requirement.
Secondly, there is another limitation that will not allow increasing the speeds of UNB systems to the level of LoRa while maintaining their other characteristics - this is the above-mentioned Shannon limit:
S = W Ă— log 2 (1 + P / WN 0 ) , where
If we substitute concrete numbers into this equation, we see that with a SNR of 60 dB, the UNB system is limited by a speed of 2 kbit / s, LoRa also goes into megabits (of course, such speeds are not implemented in practice, so in fact this result means that, within the framework of the technologies used, there are no theoretical speed limits on this side in LoRa).
In practice, this leads to the fact that adaptive speeds in UNB in ​​the case of Sigfox are not implemented at all, and in the case of Strizh, it’s traditionally impossible to understand how and how it is implemented there: representatives of the company here, on GT, write that adaptive speeds There is, but on the official website for devices, including the base stations themselves, a fixed speed of 100 bps is indicated, in the comparison plate with LoRa there is also 25600 bps, but here Shannon, Kotelnikov and Hartley clearly convey warm greetings and aggressively asked to cut sturgeon.
Immunity in UNB-systems and in LoRa
Separate attention should be paid to the question of the noise immunity of ultra-narrowband and broadband systems - if only because this is the only area where UNB-systems really have an advantage over LoRa.
In general, the main thing that needs to be understood is that
any civilian radio communication can be relatively easily drowned out (generally speaking, the military one too, but usually without the word “easy” simply because of the other powers and ranges used).
LoRa is quite sensitive to broadband interference: for example, two LoRa base stations operating side by side on the same frequency will interfere with each other quite strongly. At the same time, LoRa's sensitivity to narrowband interference is often exaggerated - in practice, LoRa has coding redundancy sufficient to safely experience its proximity to narrowband systems, including systems with pseudo-random frequency tuning. You can drown out a narrow-band system that aired a continuous stream of messages, and I even know some precedents how companies promoting such systems tried to arrange such jamming in comparative tests, but this was obviously not a case of
full- service narrow-band system, and should be considered primarily in the light of the Civil Code. Not to mention that such work of one UNB-system will create problems for other UNB-systems, if such work in the same range - even if it is not possible to drown them completely, the percentage of correctly delivered messages will fall sharply.
However, if there is a strong interference in the air, LoRa has nowhere to go from it. At the same time, the subscriber unit of the UNB-system has the opportunity to be tuned to fifty kilohertz in any direction - due to the general uncertainty described above with a specific operating frequency, the base station will still catch its signal. In particular, in UNB-systems it is possible to effectively implement the listen-before-talk scheme: a device listens to the air before turning on the transmitter, if it is too noisy in its band, it switches to transmit to another band. This scheme will not work if the noise source is located far from the device and close to the BS, but in many cases it may help. However, is this scheme implemented at all in the same Strizhe and, if so, how is the question open, the company does not respond to it on its website.
In general, it should be noted that the immunity of long-distance communication systems for IoT is a question not only technical, but also political. The situation when large communication systems are built in a low-regulated, unlicensed range is attractive from the point of view of their early implementation, but cannot continue indefinitely - the license-free ranges are effective only as long as there are not too many people willing to take on the air. It is very likely that over the course of several years - as global IoT networks evolve - changes in spectrum distribution will begin to be discussed in order to allocate licensed frequencies for such networks and thereby both simplify their deployment and avoid direct sabotage by one operator their competitors. In this case, the license-free range will remain for projects of the scale of the object - where the network is at the complete disposal of the owners of this object, and therefore does not compete with anyone.
Separately adds fuel to the fire and the fact that UNB-systems are considered very aggressive to their neighbors on the air - with active work, they strongly affect the stability of any broadband communication systems. At the same time, the developers of UNB-systems prefer to close their eyes to this problem according to the principle “who does not hide, is to blame himself”.
Instead of an afterword

This, of course, is a very rough tablet, comparing only certain aspects of the three commercially available technologies at present. In reality, the topic of long-range IoT communications is as popular as it is immense - one can talk about its various aspects, from radio planning to software, for a long time.
At the same time, the understanding of these aspects among system integrators, that potential customers of such systems are still extremely low and is based mainly on bravura press releases of some companies about how they already serve tens of thousands of square kilometers and whole million-plus cities In fact, not a single company in Russia currently has a living large projects in this area).I plan to live the same topics live at the upcoming Highload ++ 2016 conference , in which IoT section appeared this year.If your company is interested both in educational program on modern wireless IoT-technologies, and in cooperation in the development of end devices for 6LoWPAN and LoRa networks and in the elaboration of projects based on them - contact. We can do this a little bit.Unwired Devices develops and manufactures communication modules for 6LoWPAN cellular networks and LoRa long-distance networks, as well as sensors and other terminals for these networks, including both hardware and firmware, supporting the necessary network technologies. In the case of LoRa networks, we develop all possible topologies: cellular and static radio relay networks, star-based object networks from a single BS, and devices for LoRaWAN global networks.