The diversity of natural phenomena is so great, and the treasures hidden in the heavens are so rich that, thanks to their number, the human mind will never need to be fed.
- Johann Kepler
So said the man who discovered in 1604 the latest supernova at that time, located in our Galaxy and observed in the visible spectrum. And although, most likely, after it there were two more explosions, they could not be seen with the naked eye, and their remains were discovered with the help of powerful telescopes.
In January 2012, the first supernova in that year was discovered, in a galaxy 25 million light-years distant from us, NGC 3239. The supernova pictured below was named SN 2012a.

')
With a typical periodicity of about one supernova in one galaxy in one hundred years, it becomes interesting what we would see — and how quickly — if a supernova formed in our galaxy.
Recall that a supernova can be produced in one of two ways, but both of them include an out of control nuclear fusion reaction, releasing enormous amounts of light and energy. Most of the energy, surprisingly, does not stand out in the form of light! Let's look inside the star, which in a few seconds should turn into a supernova.

In addition to shocks and high temperatures, internal reactions produce neutrinos, of which most do not interact with the outer layers of the star! Only some neutrinos interact with them, as well as all protons, neutrons and electrons, the appearance of which does not occur instantly. And although the blast wave takes a couple of hours to reach the outer layers of a star, neutrinos make this journey almost instantly!
This means that when a star turns into a supernova, a stream of neutrinos occurs before the stream of light! We discovered this in observations in 1987.

When the 1987a supernova exploded just 168,000 light-years away, it was close enough — and we had enough neutrino detectors — to detect 23 (anti) neutrinos in a period of 13 seconds. The largest detector, Kamiokande II, containing 3,000 tons of water, detected 11 antineutrinos.
Today, the Super Kamiokande-III detector in its place contains 50,000 tons of water and 11,000 photomultipliers. (There are many other excellent neutrino detectors in the world, but I’ll stop here for an example).

His device is surprising because it can not only detect neutrinos, but also determine the direction, energy and point of interaction of even a single neutrino, which was lucky to interact with any of the particles in 50,000 tons of water!

Depending on where in our Galaxy a potential supernova appears, Super Kamiokande III will have to register from several thousand antineutrinos (in the event of an explosion from the opposite side of the Galaxy) to more than ten million, and all this in 10-15 seconds!
Neutrino detectors around the world will see a neutrino flow simultaneously from the same side. At this point, we will have 2-3 hours to determine the direction to the source of these neutrinos, and turn the telescopes to try to visualize the supernova - for the first time in history - from its very beginning!

The supernova nearest to 1987 was the one shown above, and we managed to see it half a day after the explosion.
Mainly due to the happy occasion, we got pretty close to the intense hypernova in 2002.

Still, we began to observe this star, SN 2002ap, only 3-4 hours after the first explosion. If a supernova that is to appear will belong to category Ia — that is, to descend from a white dwarf — we cannot predict in which part of the galaxy this will occur. There are too many white dwarfs, the location of most of them is unknown and it is believed that they are scattered throughout the galaxy.
If a supernova happens in a very massive star with a core collapsing under its own weight (type II supernova), we have a lot of good candidates for this and excellent places to look.

The obvious place is the center of the Galaxy, where the last known supernova of the Milky Way exploded, as well as the location of the most massive stars existing in our Galaxy. Over the next 100,000 years, there will definitely be a lot of type II supernovae, but we have no way of knowing when we will see the next one. Looking at the picture above, think about the fact that the explosions of these supernovae most likely have already occurred, and we are just waiting for the moment when the neutrinos (and the light behind them) reach us!
But we have candidates closer to the galactic center.

Let's look into the depths of the huge nebula in which stars are born, and find the hottest and youngest stars among all that can be found in the Universe. It is there that ultramassive stars live - and, in particular, the Eagle Nebula in the photo above can be home to a very recent supernova. The Eagle Nebula, the Orion Nebula and many other regions filled with young stars serve as excellent places for the birth of the next supernova.
What about individual stars? Although there are many good candidates, two of them especially often participate in our conversations.

This carina, which is in the very last stages of life, can literally at any moment become a supernova. Or up to this point hundreds, thousands and tens of thousands of years can pass. But if we find a stream of antineutrinos coming from about its position in space, then we will direct our telescopes to it first of all!
Unlike candidates located at distances of thousands of light years from us, there is one more, much closer. This is the closest candidate for supernova!

Say hello to Betelgeuse, the red supergiant 640 light years away. Betelgeuse is so huge that its diameter is comparable to the orbit of Saturn! If Betelgeuse turns into a supernova, our neutrino detectors throughout the Earth will register about hundreds of millions of antineutrinos, which in total will surpass the number of all neutrinos of all types ever recorded in history.
But if not these well-known candidates become supernovae, can we tell if it was a Type Ia or Type II supernova?

You can always wait. Supernovae of different types have very different light curves, and how the light fades after reaching peak brightness will show us what type of supernova it was.
But in this surprising case, I am not going to test my patience. Fortunately, I will not need it, because a supernova in our galaxy is likely to be the first recorded observation of the newest type of astronomy: astronomy of gravitational waves!
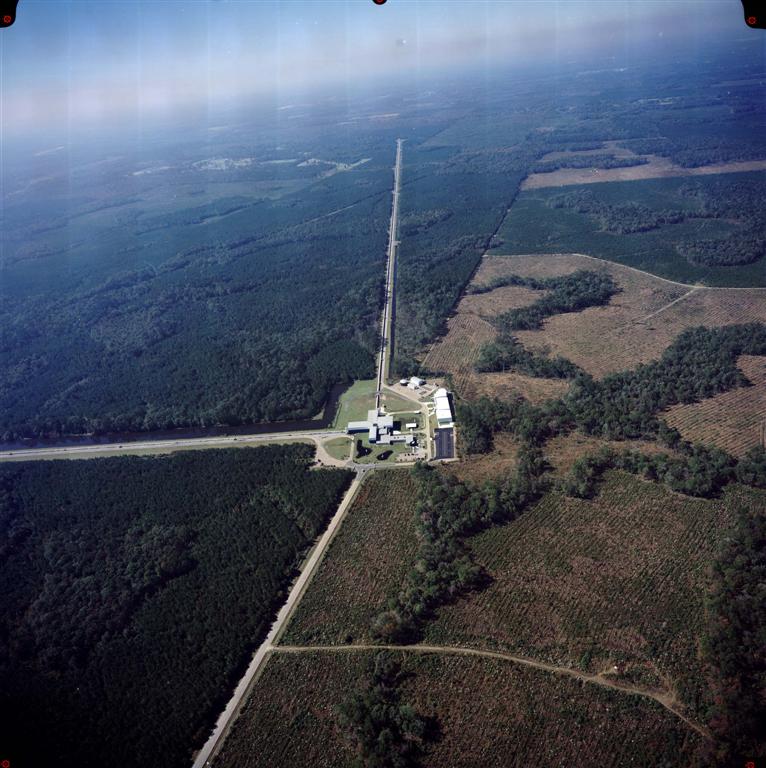
Gravitational waves do not affect anything, and such waves from a supernova explosion will have to pass through the stars, gas, dust, or matter that are in their way without disturbance, and arrive at the same time as the first wave of (anti) neutrino! And the plus will be that, according to our best GTR simulations, supernovae of type II (core collapse) and type Ia (white dwarf falling in a spiral) will have to generate completely different gravitational waves!
If this is a type Ia supernova, we will have to register three separate regions in the signal:

The spiral fall phase will have to produce a periodic pulsation, increasing frequency and strength as the white dwarfs reach the final stage of separation. At the moment of ignition, a burst should occur in the signal, followed by a decay phase. Very different things.
But if we have a type II supernova, from a supermassive collapsing star, we will see only two interesting things.

A huge burst — the supernova itself — one tenth of a second after the collapse of the nucleus, followed by a rapidly decaying (within 0.02 sec) response. And if we need to understand what we have seen, we need only such a talking signal of gravitational waves.
That's what we would see if the next supernova in our galaxy exploded today!