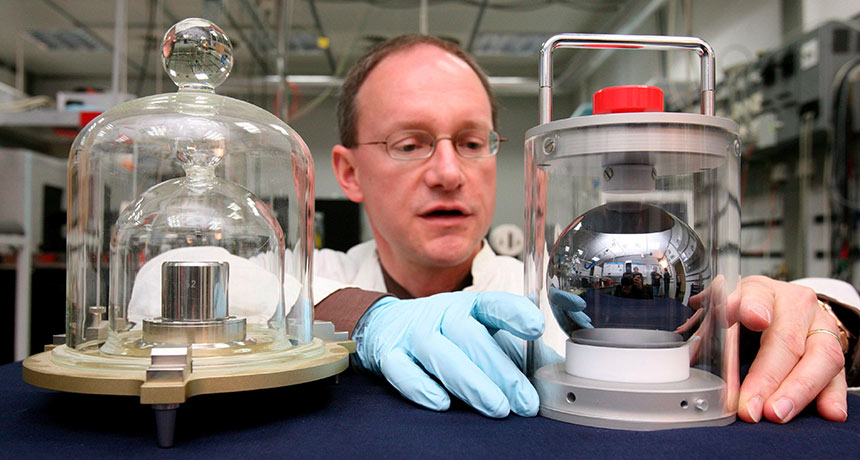
If scientists have sacred objects, then this is one of them: the only, carefully guarded 137-year-old metal cylinder located in the basement near Paris. This prototype accurately determines the value of a kilogram of mass throughout the world.
A kilo of beef at the grocery store has the same weight as this particular piece of metal, platinum and iridium. A 60-pound woman weighs 60 times more than his. Even distant astronomical objects, such as comets, are measured relative to this cylinder. Comet Churyumov-Gerasimenko, which the European Rosette spacecraft recently visited, has a mass of 10 trillion such cylinders.
But there is nothing special about this piece of metal, and its mass is not even an ideal constant. Scratches, accumulating dirt, can slightly change its mass. After that, a kilogram of meat will weigh slightly more or less than before. For hamburgers, this difference will be insignificant, but for scientific measurements a small shift in the definition of a kilogram can lead to big problems.
')
This topic worries some researchers. They would prefer to define important units of measurement - kilograms, meters, seconds - through the unchanging properties of reality, rather than at random taken lengths, masses and other quantities, invented by scientists. If humans had met aliens and compared the measurement systems of each other, as physicist Stephan Schlamminger says, “the whole Galaxy would laugh at us”.
To remedy the situation,
metrologists — a rare type of scientist engaged in accurate measurements — correct the system. Soon they will begin to use the fundamental constants of nature — numbers that do not change, such as the speed of light, the electron charge, and Planck's constant — to calibrate their lines, weights, and thermometers. They have already got rid of the artificial definition of a meter - a bar of platinum and iridium with engraving. In 2018, they will throw out the Paris kilogram cylinder.
In 2018, the seven units of the international system of units (inner circle) will be redefined through seven constants (outer circle).Fundamental constants are among the most accurately measured parameters, so they seem ideal for determining units of measurement. But they themselves are mysteries. The fine structure constant serves as a puzzle for physicists since its appearance in the equations 100 years ago. Every time when electrically charged particles attract or repel each other, anywhere in the Universe, this constant plays its role. Its value determines the force of repulsion and attraction. Change it by a few percent - and the stars will begin to create less carbon, the foundation of all life. A little bit more - and the stars, molecules and atoms do not arise at all. One gets the impression that its value was chosen specifically for life in the Universe.
The values of other fundamental constants also cannot be explained - one can only measure. “No one knows why these constants have such values,” says theoretical physicist John Barrow from the University of Cambridge.
This uncertainty surrounding the constants may cause inconvenience to metrologists. The laws of physics do not forbid constants to vary in time or space — although no evidence of such changes was found. Some controversial measurements hint that the fine structure constant may be different in different parts of the Universe. This may mean that other constants change, and this will destroy the entire neat system, which metrologists are preparing to adopt.
Collection of constants
Constant | Symbol | Physical sense | Value | Defines |
---|
Planck's constant | h | Defines the scope of quantum mechanics | 6.626070040 × 10 −34 kg * m 2 / s | kilogram |
The speed of light in a vacuum | c | Maximum object speed | 299 792 458 m / s | meter |
Elementary charge | e | Electric charge of electron and proton | 1.6021766208 × 10 −19 ampere * seconds | ampere |
Boltzmann's constant | k | Energy conversion to temperature | 1,38064852 × 10 −23 kg * m 2 / (s 2 * K) | kelvin |
Permanent Avogadro | N a | The number of particles in one mole of matter | 6.022140857 × 10 23 / mol | mole; together with R ∞ determines h and then kilogram |
Rydberg constant | R ∞ | Determines the wavelength of light emitted by a hydrogen atom. | 10,973,731.568508 / m | kilogram; together with N A determines h and then kilogram |
Ultrafine Cesium Cleavage | ∆ν Cs | Cesium atomic clock frequency | 9,192,631,770 / s | second |
Light efficiency | K cd | Converting brightness to power | 683 candela * steradian * c 3 / (kg * m 2 ) | candela |
Fine structure constant | α | The strength of the electromagnetic interaction between charged particles | 1 / 137,035999139 | used with other constants |
Hair crack
Scientists are not only a kilogram of poor health. The next accused is Kelvin, the unit of measure for temperature.
“She’s crazy,” says physicist Michael de Podesta from the State Physical Laboratory in Teddington, England. "Human temperature standards are the level of jitter of molecules at a strange point." This point is similar to the sacred kilogram chosen at random - a triple point of water, a certain temperature and pressure at which the liquid, gaseous and solid phases of water coexist. This temperature is assigned a value of 273.16 Kelvin (0.01 ° C).
And there is also an ampere denoting the flow of electricity that powers laptops and light bulbs. “We have suffered for years with the definition of an amp,” said Barry Inglis, president of the International Committee for Weights and Measures. The current weak definition is as follows: this is a flow that, when flowing through two infinitely long and infinitely thin wires located one meter apart, will result in the interaction of a certain force between them. But since such wires cannot be made, in practice it is not very convenient to determine amperes in this way. As a result, measuring equipment is difficult to calibrate. This is not a problem for electricians pulling in wiring in your home, but in terms of high accuracy measurements, this is not good.
These examples show the level of discomfort surrounding the fundamental units of measure that are so important to science. “There was a hairline in the foundation, and on this basis the building of physics cannot be built,” says Schlamminger of the National Institute of Standards and Technology in Gettysburg.
To seal the crack, scientists want to update the international system of units of measurement, or SI, in 2018. Kilogram, kelvin, ampere and mole (units that measure the amount of a substance) will be redefined using constants associated with them. This will include the Planck constant, which determines the scale of the quantum world; Boltzmann constant that relates temperature to energy; Avogadro's constant specifying the number of atoms or molecules in a mole; the amount of charge of an electron or proton, also known as elementary charge. The new units will be based on the modern understanding of physics, including the laws of quantum mechanics and Einstein's special theory of relativity.
Scientists have already engaged in such exercises, redefining the meter through a fundamental constant - the speed of light, which is always the same.
In 1983, the meter turned into the distance that light travels in vacuum for 1/299 792 458 a split second. This sequence of numbers came from the constantly updated speed of light. Scientists have agreed to consider it equal to exactly 299 792 458 meters per second, which determines the meter. Other units will undergo similar changes.
Shaking up so many units happens “once in a lifetime,” explains physicist David Newel from the National Institute of Standards and Technology. But most people will not notice. The definitions will change, but the changes will take place so that the dimensions of the kilogram or kelvin do not change. You do not have to overpay for a salad bar.
And although the changes will mostly be hidden, their benefits are not only philosophical. In the current system, it is difficult to measure masses that are very different from kilograms. Pharmacists have to measure small fractions of grams for the manufacture of medicines. They can be one millionth of a kilogram cylinder, which increases the measurement inaccuracy. The new system will tie the mass to the Planck constant, which will allow more accurate measurements of both large and small masses.
In 2018, at the General Conference of Weights and Measures, metrologists will vote for changes in the SI and will most likely accept them. It is expected that the new system will accept with joy. “Obviously, a system in which you can take a lump of metal and declare“ this is a kilogram ”is not very fundamental,” says physicist Richard Davis from the International Bureau of Weights and Measures in France. “Who will spend their lives trying to measure the atom in this way?”
New kilogram
To retire the Paris prototype, scientists must agree on the value of Planck's constant. It is about 6.62607 x 10
−34 kg * m
2 / s. But it must be measured with particular accuracy - up to 2 millionths of a percent, that is, up to seven decimal places - and the various measurements must match. After this, the value of the constant will be changed. Since the meter is already determined by the speed of light, and the second is determined by the cesium atomic clock, the change in Planck's constant will be determined by the kilogram.
Scientists use watt-scales , arranging a contest between electromagnetism and gravity to measure Planck's constant. Watt-scales on a photo are made by Stefan Schlamminger.Several teams use different measurement techniques. The first compares electromagnetism with gravity using watt weights. The Schlamminger Group is in the final stages of setting up the tool. Due to exact quantum-mechanical methods of obtaining stresses, the mass of an object can be directly compared with the Planck constant.
Others measure a constant through extremely shiny silicon balls made with great precision. “The principle is simple,” says metrologist Horst Bettin of the German National Institute of Metrology, Physikalisch-Technische Bundesanstalt. “We just count the number of atoms.”
Atoms in a sphere are evenly located in an ideal three-dimensional lattice, so their number can be calculated from the size of the sphere. The resulting measurement of the Avogadro constant can be used to calculate Planck’s constant, by accurately measuring other fundamental constants — including the Rydberg constant related to the ionization energy of a hydrogen atom. For such measurements, the spheres need to be perfectly round so that the number of their atoms can be counted. “The earth could be compared in roundness with our spheres if the highest of the mountains did not exceed a few meters,” says Bettin.
Questioning constants
Imagine a universe in which the speed of light varies greatly from day to day. If a modern metrological system were used there, then “today's meter would be different from tomorrow's one,” says Schlamminger, which is clearly an imperfect situation. No evidence of such variability has been found in our Universe, and if it exists in very small limits, it will not have a practical impact on the measurement system.
But if the constants were not constant, physicists would have a hard time. All physics is based on the assumption of immutability of laws, says physicist Paul Davies [Paul Davies] of Arizona State University.
Physicists have found signs of possible inconstancy in the fine structure constant. If so, it turns out that the charged particles behave differently in different parts of the universe.
The fine structure constant is the fusion of several other constants, including the electron charge, the speed of light and Planck's constant, and their mixture results in a number approximately equal to 1/137. This is an off-system unit, therefore its value does not change in different measurement systems.

Scientists track it through quasars - amazing space beacons, arising from remote supermassive black holes. On the way to the earth, the light of a quasar passes through clouds of gas, which absorb light at certain frequencies, which leads to the appearance of gaps in the spectrum of light. The position of the gaps depends on the fine structure constant. Changes in the position of gaps in time or space can mean changes to this constant.
In 2011, scientists reported provoking hints of constant changes. Astrophysicist John Webb of the University of New South Wales and his colleagues reported that the fine structure constant increases in one direction of the celestial sphere, and decreases in the other direction, as if there is a special axis in the Universe. The statement is controversial, and Webb himself refers to the skeptics. "This, of course, is radical, and when you make such a discovery, you do not believe it." But, despite all the attempts to refute this evidence, the variability remains in place.
If the observations are confirmed, the consequences will be incredible. “The effect is very small,” says Davis, “but I think it will be a shock, because people want the laws of physics to be unchanged. The idea of their variability is of great concern to most physicists. ”
Some scientists have come up with calming explanations for the variability of the constant. Michael Murphy of Swinburne University of Technology in Melbourne suggests that this is the problem with telescope calibration. Murphy co-authored the work of 2011, which reported variations in the constant. In September, Murphy and colleagues reported that, when using a telescope without calibration problems, confirms the invariance of the fine structure constant. But the quasars described in this paper do not exclude the presence of changes in that part of the sky, which was studied for work from 2011.
The possibility of changing the constants can be attributed to other mysteries of physics. Scientists believe that invisible dark matter makes up most of the substance of the universe. In their work in 2015, physicists Victor Flambaum and Eugene Stadnik from the University of New South Wales showed that dark matter can change fundamental constants by interacting with ordinary matter.
Changes in the speed of light can affect modern ideas about the evolution of the Universe. Scientists believe that during inflation the space expanded extremely rapidly, which led to the emergence of a uniform universe on a large scale. This is consistent with observations: the temperature of the background radiation, or light, which arose 380,000 years after the Big Bang, is almost the same everywhere. But the cosmologist João Magueijo from Imperial College London offers an alternative to inflation: if the speed of light were higher in the early Universe, this would explain its uniformity.
“If we raise the speed of light in the early Universe,” he says, “there is an opportunity to work on explaining its current state.”
Fine tuning universe
To the horror of many physicists whose equations are riddled with fundamental constants, these values cannot be derived from physical principles. Scientists do not know why electrons are attracted to charged particles with just such a force, and can only measure its value and put it into formulas. Such black boxes diminish the elegance of scientific theories, trying to explain the universe from beginning to end.
Especially exciting is the fact that the exact values of these constants are extremely important for the appearance of stars and galaxies. If at the time of the birth of the universe certain constants — in particular, the fine structure constant — would be a little different, then the cosmos would be empty and sterile.
As a result, many believe that there should be some theory that limits these values. But recent attempts at developing this theory have stalled, says theoretical physicist Frank Wilczek of MIT. "Progress has been slow over the past decades."
Some scientists turned to an alternative explanation: the constants may not be specially selected, but they were chosen randomly, and these dice rolls occurred many times in many universes, or in different parts of the Universe. “We changed the concept of fundamental constants. They are not so tightly defined and final, ”says Barrow.
There may be other universes, or remote parts of our Universe where other constants work. In those places, life may not survive. Just as diverse life evolved on Earth, in a suitable climate, and not on Mars, so our Universe can have constants prone to life, since only here could it originate.
The discrepancy between experimental data and theoretical calculations about the constants also increases. Although scientists measure them with incredible accuracy, and measurement errors are only billionths, the origin of the constants remains unexplained.
And although metrologists are trying to build their system on a more reliable foundation, tying the units of measurement to constants, this foundation can still sway. Changing constants will make the system less beautiful and neat. Newel says that the system of units will have to evolve with knowledge and science. "And then it will be possible to look around and use this measurement system to further explore the world around us."