Professor Andrew Traskot and student Roman Khakimov bravely peer into the quantum world.In a study on the behavior of quantum particles,
scientists from the Australian National University confirmed that quantum particles can behave so strangely that it seems that they violate the
principle of causality .
This principle is one of the fundamental laws that few people dispute. Although many physical quantities and phenomena do not change, if we reverse the time (are
T-even ), there is a fundamental empirically established principle: event A can influence event B only if event B happens later. From the point of view of classical physics - just later, from the point of view of SRT - later in any reference system, i.e., it is in the light cone with vertex in A.
')
So far, only science fiction fighters are fighting the “
paradox of the killed grandfather ” (I recall a story in which it turned out that grandfather had nothing to do with it, but it was necessary to deal with her grandmother). In physics, traveling into the past is usually associated with traveling faster than the speed of light, and so far
everything has
been calm .
Except for one moment - quantum physics. There are a lot of strange things there. For example, a classic experiment with two slits. If we place an obstacle with a slit in the path of the source of particles (for example, photons), and put the screen behind it, then we will see a strip on the screen. Is logical. But if we make two slots in the obstacle, then on the screen we will see not two stripes, but an interference pattern. Particles, passing through the cracks, begin to behave like waves, and interfere with each other.
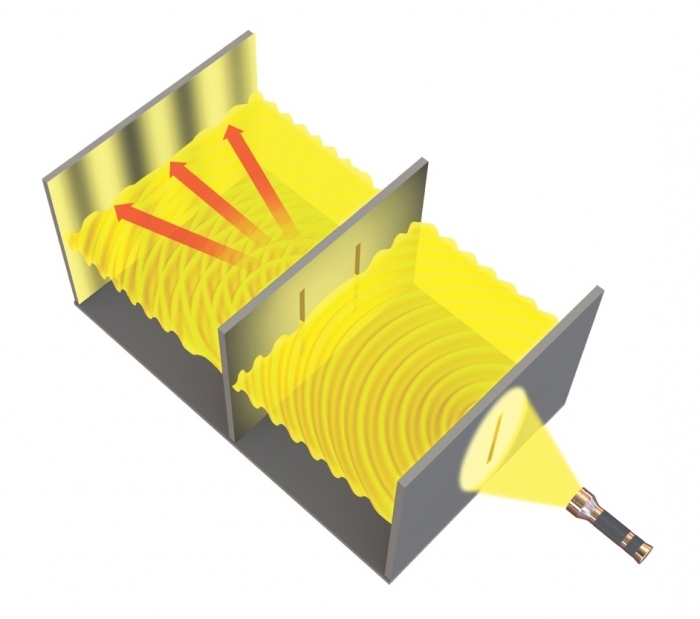
To eliminate the possibility that particles collide on the fly with each other and therefore do not draw two clear stripes on our screen, you can release them one by one. Still, after some time an interference pattern will appear on the screen. Particles magically interfere with themselves! This is much less logical. It turns out that the particle passes through two slots at once - otherwise, how can it interfere?
And then - even more interesting. If we try to understand what a slit a particle passes through, then when we try to establish this fact, the particles instantly begin to behave like particles and cease to interfere with themselves. That is, the particles practically "feel" the presence of a detector at the slits. Moreover, interference is obtained not only with photons or electrons, but even with particles that are quite large by quantum standards. To exclude the possibility that the detector somehow "spoils" the approaching particles, quite complex experiments were performed.
For example, in 2004, an experiment was conducted with a beam of fullerenes (C
70 molecules containing 70 carbon atoms). The beam was scattered on a diffraction grating consisting of a large number of narrow slits. At the same time, experimenters could controlly heat molecules flying in a beam by means of a laser beam, which made it possible to change their internal temperature (average vibration energy of carbon atoms inside these molecules).
Any heated body emits thermal photons, the spectrum of which reflects the average energy of transitions between possible states of the system. For several such photons, it is possible, in principle, up to the wavelength of the emitted quantum, to determine the trajectory of the molecule that emitted them. The higher the temperature and, accordingly, the smaller the wavelength of the quantum, the more accurately we could determine the position of the molecule in space, and at a certain critical temperature the accuracy would be sufficient to determine at which particular gap the scattering occurred.
Accordingly, if someone surrounded the installation with perfect photon detectors, he could, in principle, establish at which of the slots of the diffraction grating fullerene scattered. In other words, the emission of light quanta by a molecule would give the experimenter the information for separating the components of the superposition that the transit detector gave us. However, there were no detectors around the installation.
In the experiment, it was found that in the absence of laser heating, an interference pattern is observed that is completely analogous to the picture from two slots in the experiment with electrons. The inclusion of laser heating leads first to a weakening of the interference contrast, and then, as the heating power increases, to the complete disappearance of the effects of interference. It was found that at temperatures T <1000 K, molecules behave like quantum particles, and at T> 3000 K, when the trajectories of fullerenes are “fixed” by the environment with the necessary accuracy, like classical bodies.
Thus, the role of a detector capable of distinguishing components of a superposition turned out to be able to fulfill the environment. It interacts with thermal photons in one form or another and recorded information about the trajectory and state of the fullerene molecule. And it doesn’t matter at all what information is being exchanged: through a specially delivered detector, the environment or a person.
For the destruction of the coherence of the states and the disappearance of the interference pattern, only the fundamental availability of information matters, which of the slits the particle went through - and who will receive it, and whether it is received, is no longer important. It is only important that such information is fundamentally possible to obtain.
It seems to you that this is the strangest manifestation of quantum mechanics? As if not so. Physicist John Wheeler proposed a thought experiment in the late 1970s, which he called the "
experiment with a deferred choice ." His reasoning was simple and logical.
Well, let us assume that a photon finds out in some unknown way that it will or will not be tried to be detected, before approaching the slots. After all, he needs to somehow decide - to behave like a wave, and go through both slots at once (in order to later meet the interference pattern on the screen), or pretend to be a particle, and go through only one of the two slots. But he needs to do this before he goes through the cracks, right? After that, it's too late - either fly there, like a small ball, or interfere with the full program.
So let's, Wheeler suggested, place the screen away from the cracks. And behind the screen we will install two telescopes, each of which will be focused on one of the slits, and will only respond to the passage of a photon through one of them. And we will arbitrarily remove the screen after the photon passes the gap, no matter how he decided to go through them.
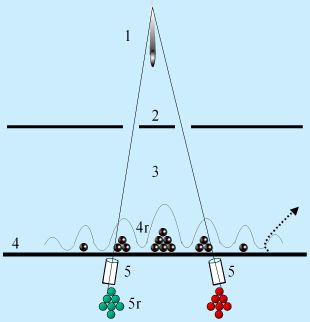
If we do not remove the screen, then in theory, it should always be a picture of interference. And if we remove it - then either the photon will fall into one of the telescopes, like a particle (it went through one slit), or both telescopes will see a weaker glow (it went through both slits, and each of them saw his own part of the interference pattern) .
In 2006, progress in physics allowed scientists
to set up such an experiment with a photon in fact. It turned out that if the screen is not removed, the picture of interference is always visible on it, and if it is removed, then it is always possible to track which slit the photon went through. Arguing from the point of view of the logic familiar to us, we arrive at a disappointing conclusion. Our action on deciding whether or not we remove the screen influenced the behavior of the photon, despite the fact that the action is in the future with respect to the photon's “solution” on how to pass the slits. That is, either the future influences the past, or there is something fundamentally wrong in the interpretation of what is happening in the gap experiment.
Australian scientists have repeated this experiment, only instead of a photon, they used a helium atom. An important difference of this experiment is the fact that an atom, unlike a photon, has a rest mass, as well as different internal degrees of freedom. Only instead of an obstacle with slits and a screen, they used grids created with the help of laser beams. This enabled them to immediately receive information about the behavior of the particle.
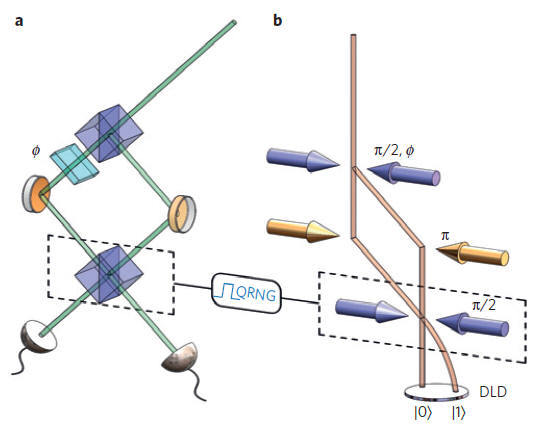
As one would expect (although it is hardly worth expecting anything with quantum physics), the atom behaved just like a photon. The decision on whether or not there will be a “screen” in the path of the atom was made on the basis of the work of a quantum random number generator. The generator was by relativistic standards shared with the atom, that is, there could be no interaction between them.
It turns out that individual atoms that have mass and charge behave in the same way as individual photons. And let this not the most breakthrough experience in the quantum field, but it confirms the fact that the quantum world is not at all the way we can imagine it.