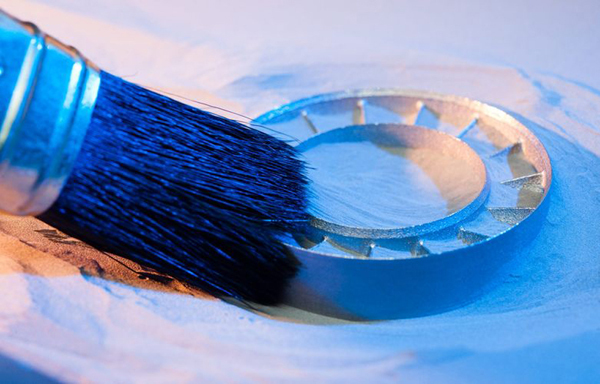
In this review, I tried in a popular form to provide basic information about the production of metal products using laser additive manufacturing - a relatively new and interesting technological method that emerged in the late 80s and has become today a promising technology for small-scale or individual production in the field of medicine, aircraft - and rocket science.
Briefly describe the principle of operation of the installation for additive production using laser radiation can be as follows. A device for applying and leveling a layer of powder removes a layer of powder from the feeder and spreads it evenly over the surface of the substrate. After that, the laser beam scans the surface of this powder layer and forms a product by melting or sintering. At the end of the scanning of the powder layer, the platform with the product being manufactured is lowered by the thickness of the applied layer, and the platform with the powder rises, and the process of applying the powder layer and scanning is repeated. After the process is completed, the platform with the product is lifted and cleaned of unused powder.

One of the main parts in an additive manufacturing facility is a laser system using CO
2 , Nd: YAG, ytterbium fiber or disk lasers. It has been established that the use of lasers with a wavelength of 1-1.1 μm for heating metals and carbides is preferable, since they are 25-65% better absorbed by the laser-generated radiation. At the same time, the use of a CO
2 laser with a wavelength of 10.64 microns is best suited for materials such as polymers and oxide ceramics. Higher absorption capacity allows to increase the depth of penetration and in a wider range to vary the process parameters. Usually lasers used in additive manufacturing work in a continuous mode. In comparison with them, the use of lasers operating in a pulsed mode and in a modulated Q-factor due to their high pulse energy and short pulse duration (nanoseconds) makes it possible to improve the bond strength between the layers and reduce the heat-affected zone. In conclusion, it can be noted that the characteristics of the laser systems used lie within the following limits: laser power - 50-500 W, scanning speed up to 2 m / s, positioning speed up to 7 m / s, focused spot diameter - 35-400 microns.
')
In addition to the laser, electron beam heating can be used as a source of heating the powder. Arcam offered this option and implemented it in its installations in 1997. The installation with an electron-beam gun is characterized by the absence of moving parts, since the electron beam is focused and directed using a magnetic field and deflectors, and the creation of a vacuum in the chamber has a positive effect on the quality of the products.
One of the important conditions for additive manufacturing is the creation of a protective environment that prevents the oxidation of the powder. Argon or nitrogen is used to fulfill this condition. However, the use of nitrogen as a protective gas is limited, which is connected with the possibility of the formation of nitrides (for example, AlN, TiN in the manufacture of products from aluminum and titanium alloys), which lead to a decrease in the plasticity of the material.
Laser additive manufacturing methods can be divided into Selective Laser Sintering (SLS), indirect laser sintering of metals (Indirect Metal Laser Sintering (IMLS)), and direct laser sintering of metals (Direct Metal Laser Sintering (DMLS) ) and selective laser melting (Selective Laser Melting (SLM)). In the first embodiment, the compaction of the powder layer occurs due to solid-phase sintering. In the second, due to the impregnation with a bunch of porous framework previously formed by laser radiation. The direct laser sintering of metals is based on the compaction by the mechanism of liquid-phase sintering due to the melting of the low-melting component in the powder mixture. In the latter case, compaction occurs due to complete melting and spreading of the melt. It is worth noting that this classification is not universal, since in one type of additive manufacturing process, sealing mechanisms may occur, which are characteristic of other processes. For example, with DMLS and SLM, solid phase sintering can occur, which occurs with SLS, while with SLM, liquid phase sintering can occur, which is more characteristic of DMLS.
Selective Laser Sintering (SLS)
Solid-phase selective laser sintering is not widely used, since for a more complete flow of bulk and surface diffusion, viscous flow and other processes that occur during sintering of the powder, relatively long exposure is required under laser radiation. This leads to long-term laser operation and low productivity of the process, which makes this process not economically viable. In addition, there are difficulties in maintaining the process temperature in the interval between the melting point and the temperature of solid-phase sintering. The advantage of solid-phase selective laser sintering is the possibility of using a wider range of materials for the manufacture of products.
Indirect Laser Metal Sintering (IMLS)
The process, called "indirect laser sintering of metals" was developed by DTMcorp of Austin in 1995, which since 2001 has been owned by 3D Systems. In the IMLS process, a mixture of powder and polymer or a powder coated with a polymer is used, where the polymer acts as a binder and provides the necessary strength for further heat treatment. At the stage of heat treatment, the polymer is distilled off, the frame is sintered and the porous frame is impregnated with a metal ligament, as a result of which the finished product is obtained.
For IMLS, powders can be used, both metals and ceramics or their mixtures. Preparation of a mixture of powder with a polymer is carried out by mechanical mixing, while the content of the polymer is about 2-3% (by weight), and in the case of using the powder coated polymer, the layer thickness on the surface of the particle is about 5 microns. Epoxies, water glass, polyamides and other polymers are used as a bond. The temperature of the distillation of the polymer is determined by the temperature of its melting and decomposition and is on average 400-650
o C. After the distillation of the polymer, the porosity of the product before impregnation is about 40%. During impregnation, the furnace is heated 100-200
° C above the melting point of the impregnating material, since with increasing temperature the wetting angle decreases and the melt viscosity decreases, which favorably influences the impregnation process. Usually, future products are impregnated in an alumina bed, which plays the role of supporting the carcass, since in the period from the distillation of the polymer to the formation of strong interparticle contacts there is a danger of destruction or deformation of the product. Protection against oxidation is organized by creating inert or reducing media in a furnace. For impregnation, you can use quite a variety of metals and alloys that satisfy the following conditions. The impregnation material should be characterized by a complete absence or a slight interfacial interaction, a small wetting angle and have a melting point lower than that of the base. For example, if the components interact with each other, then during the impregnation process undesirable processes may occur, such as the formation of more refractory compounds or solid solutions, which can lead to a stop of the impregnation process or adversely affect the properties and dimensions of the product. Usually bronze is used for the impregnation of the metal frame, while the shrinkage of the product is 2-5%.

One of the drawbacks of IMLS is the inability to regulate the content of the refractory phase (base material) over a wide range. Since its percentage in the finished product is determined by the bulk density of the powder, which, depending on the characteristics of the powder, can be three or more times less than the theoretical density of the powder material.
Materials and their properties used for IMLS
Direct laser sintering of metals (DMLS)
The process of direct laser sintering of metals is similar to IMLS; however, it differs in that instead of a polymer, alloys or compounds with a low melting point are used, and there is no such technological operation as impregnation. The basis for creating the DMLS concept was the German company EOS GmbH, which in 1995 created a commercial installation for the direct laser sintering of a steel-nickel bronze powder system. The production of various products using the DMLS method is based on the flow of the formed melt-bundle into the voids between the particles under the action of capillary forces. At the same time, to successfully complete the process, compounds with phosphorus are added to the powder mixture, which reduce surface tension, viscosity and degree of melt oxidation, thereby improving wettability. The powder used as a binder is usually smaller than the base powder, as this allows the bulk density of the powder mixture to be increased and the melt formation process to be accelerated.
Materials and their properties used for DMLS by EOS GmbH
Selective Laser Melting (SLM)
Further improvement of facilities for additive manufacturing is associated with the emergence of the possibility of using a more powerful laser, a smaller diameter of the focusing spot and a thinner layer of powder, which allowed the use of SLM for the manufacture of products from various metals and alloys. Usually the products obtained by this method have a porosity of 0-3%
As in the above discussed methods (IMLS, DMLS), the wettability, surface tension and melt viscosity play a large role in the process of manufacturing products. One of the factors deterring the use of various metals and alloys for SLM is the effect of “ball formation” or spheroidization, which manifests itself in the form of the formation of droplets lying separately from each other, and not a solid melt path. The reason for this is the surface tension under the action of which the melt tends to reduce the free surface energy by forming a mold with a minimum surface area, i.e. ball. In this case, the Marangoni effect is observed in the melt strip, which manifests itself in the form of convective flows due to the surface tension gradient as a function of temperature, and if the convective flows are strong enough, the melt strip is divided into separate drops. Also, a melt drop under the action of surface tension draws into itself nearby powder particles, which leads to the formation of pits around the drop and, ultimately, to an increase in porosity.
Spheroidization of M3 / 2 steel in non-optimal SLM modesThe effect of spheroidization also contributes to the presence of oxygen, which dissolves in the metal, increases the melt viscosity, which leads to a deterioration of the spreading and wettability of the melt below the underlying layer. For the above reasons, it is not possible to obtain products from metals such as tin, copper, zinc, lead.
It is worth noting that the formation of a high-quality melt strip is associated with the search for the optimal process parameter region (laser power and scanning speed), which is usually quite narrow.
The effect of SLM gold parameters on the quality of the formed layersAnother factor affecting the quality of products is the appearance of internal stresses, the presence and magnitude of which depends on the geometry of the product, the rate of heating and cooling, the coefficient of thermal expansion, phase and structural changes in the metal. Significant internal stresses can lead to deformation of products, the formation of micro- and macrocracks.
Partially, the negative impact of the above factors can be reduced by using heating elements, which are usually located inside the installation around the substrate or powder feeder. Heating the powder also allows you to remove adsorbed moisture from the surface of the particles and thereby reduce the degree of oxidation.
In the selective laser melting of metals such as aluminum, copper, and gold, an important problem is their large reflectivity, which necessitates the use of a powerful laser system. But increasing the power of the laser beam can adversely affect the dimensional accuracy of the product, since if heated excessively, the powder will melt and sinter outside the laser spot due to heat transfer. High laser power can also lead to changes in the chemical composition as a result of metal evaporation, which is especially characteristic of alloys containing low-melting components and high vapor pressure.
Mechanical properties of materials obtained by the SLM method (EOS GmbH)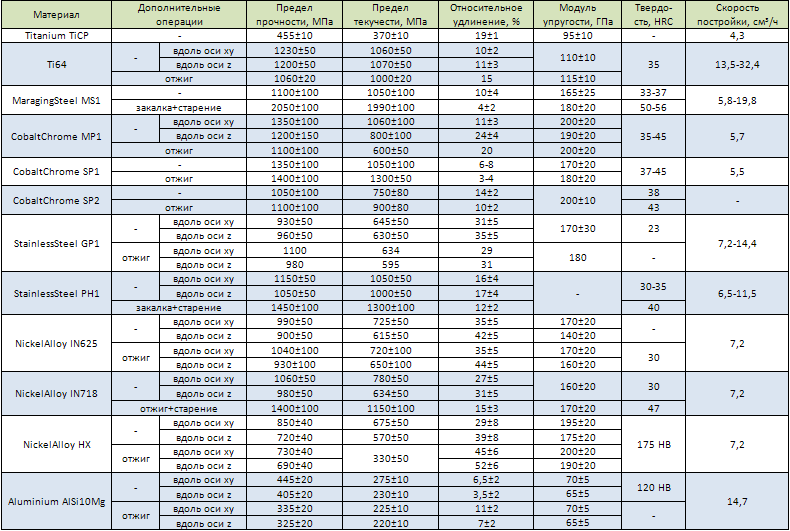
If the product obtained by one of the above methods has residual porosity, then, if necessary, additional technological operations are used to increase its density. For this purpose, powder metallurgy methods are used - sintering or hot isostatic pressing (HIP). Sintering allows you to eliminate the residual porosity and improve the physico-mechanical properties of the material. It should be emphasized that the formed material properties in the sintering process are determined by the composition and nature of the material, the size and number of pores, the presence of defects and other numerous factors. An ISU is a process in which a billet placed in a gasostat is compacted under the action of high temperature and all-round compression with an inert gas. The working pressure and the maximum temperature reached by the gas-meter depends on its design and volume. For example, a gas stove having a working chamber size of 900x1800 mm is capable of developing a temperature of 1500
o C and a pressure of 200 MPa. The use of the GUI to eliminate porosity without the use of a sealed shell is possible if the porosity is no more than 8%, since, with a larger value, the gas will penetrate through the pores into the product, thereby preventing compaction. It is possible to eliminate the penetration of gas into the product by fabricating a steel hermetic shell that follows the shape of the product surface. However, the products obtained by additive production, mainly have a complex shape, which makes it impossible to manufacture such a shell. In this case, for sealing, you can use a vacuum-sealed container in which the product is placed in a free-flowing medium (Al
2 O
3 , BN
hex , graphite), which transfers pressure to the walls of the product.
After the additive production by the SLM method, materials are characterized by anisotropy of properties, increased strength and low ductility due to the presence of residual stresses. To relieve residual stresses, to obtain a more equilibrium structure, to increase the viscosity and plasticity of the material, annealing is carried out.
According to the data below, it can be noted that the products obtained by selective laser melting, in some cases, are stronger than cast ones by 2-12%. This can be explained by the small grain size and microstructural components, which are formed as a result of rapid cooling of the melt. Rapid supercooling of the melt significantly increases the number of nuclei of the solid phase and reduces their critical size. At the same time, crystals rapidly growing on the embryos, touching each other, begin to hinder their further growth, thereby forming a fine-grained structure. Crystallization nuclei are usually non-metallic inclusions, gas bubbles or particles released from the melt, with their limited solubility in the liquid phase. And in the general case, according to the Hall-Petch ratio, with decreasing grain size, metal strength increases due to the developed network of grain boundaries, which is an effective barrier to the movement of dislocations. It should be noted that due to the different chemical composition of the alloys and their properties, the conditions of the SLM, the above-mentioned phenomena that take place during the cooling of the melt appear with different intensities.
Mechanical properties of materials obtained by SLM and casting
Of course, this does not mean that the products obtained by selective laser melting are better than products obtained by traditional methods. Due to the great flexibility of traditional methods of producing products, it is possible to vary the properties of the product over a wide range. For example, using such methods as changing the temperature conditions of crystallization, doping and the introduction of modifiers into the melt, thermal cycling, powder metallurgy, thermomechanical treatment, etc., one can achieve a significant increase in the strength properties of metals and alloys.
Of particular interest is the use of carbon steel for additive production, as a cheap and highly complex mechanical properties of the material. It is known that with increasing carbon content in steel, its fluidity and wettability are improved. This makes it possible to obtain simple products containing 0.6-1% C with a density of 94-99%, while in the case of using pure iron, the density is about 83%. In the process of selective laser melting of carbon steel, the melt path under rapid cooling is subjected to quenching and tempering on the structure of troostite or sorbitol. At the same time, due to thermal stresses and structural transformations, considerable stresses can occur in the metal, which lead to a product lead or to the formation of cracks. Also important is the geometry of the product, since sharp transitions over the cross section, small radii of curvature and sharp edges cause the formation of cracks. If, after “printing”, the steel does not have the specified level of mechanical properties and it needs to be subjected to additional heat treatment, then it will be necessary to reckon with the previously noted restrictions on the shape of the product to avoid the appearance of hardening defects. This, to some extent, reduces the prospects of using SLM for carbon steels.
When producing products using traditional methods, one of the ways to avoid cracks and leads when quenching products of complex shape is the use of alloyed steels, in which the alloying elements present, in addition to enhancing the mechanical and physicochemical properties, delay the austenite transformation during cooling, resulting in a decrease in the critical quenching rate and hardenability of the alloyed steel increases. Due to the low critical rate of quenching, steel can be rolled in oil or in air, which reduces the level of internal stresses. However, due to the rapid heat removal, the inability to control the cooling rate and the presence of carbon in the alloyed steel, this technique does not allow to avoid the appearance of significant internal stresses during selective laser melting.
In connection with the above-noted features, for SLM, martensite-straining steels (MS 1, GP 1, PH 1) are used, in which hardening and increase in hardness is achieved due to the release of dispersed intermetallic phases during heat treatment. These steels contain a small amount of carbon (hundredths percent), as a result of which the martensite lattice formed upon rapid cooling is characterized by a small degree of distortion and, consequently, has a low hardness. The low hardness and high ductility of martensite ensure the relaxation of internal stresses during quenching, and the high content of alloying elements allows the steel to be calcined to a great depth at almost any cooling rate. Thanks to this, with the help of SLM, complex products can be manufactured and heat treated without fear of cracking or warping. In addition to martensitic-aging steels, some austenitic stainless steels can be used, for example, 316L.
In conclusion, it can be noted that now the efforts of scientists and engineers are aimed at a more detailed study of the effect of process parameters on the structure, mechanism and features of compaction of various materials under the action of laser radiation in order to improve the mechanical properties and increase the range of materials suitable for laser additive production.