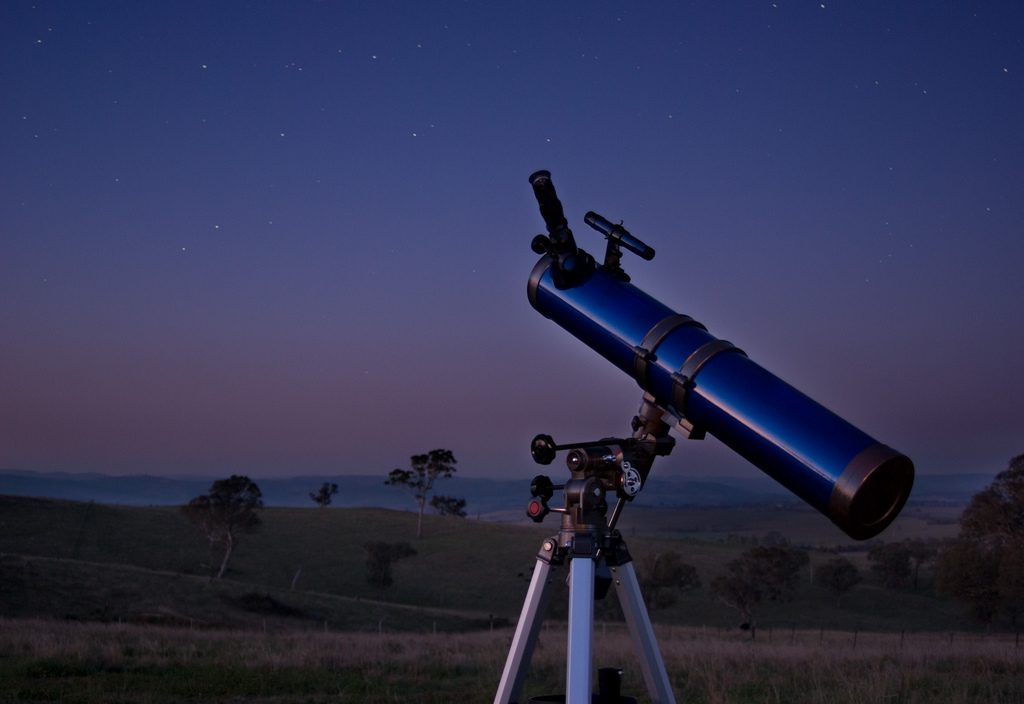
[From the translator]
Probably many, when it comes to searching for exoplanets, imagine a person looking through the eyepiece of a huge telescope. Unfortunately, this does not work. Even for the best modern telescopes, observing exoplanets is a difficult task, since the planets themselves are small in size, and their radiation is extremely difficult to distinguish from the stars. For this purpose, a number of interesting methods are used, one of which is ADI (Angular Differential Imaging) and will be discussed in this article.Introduction
One of the most difficult tasks facing modern astronomy is the shooting of planets outside the solar system and revolving around other stars. In terms of complexity, this task can be compared with the search for a firefly that flies around a 300-watt searchlight several kilometers away from an observer - in order to distinguish between nearby sources of radiation from each other, you will need not only a large-diameter mirror, but the most advanced optics able to compensate for the distortions introduced by the atmosphere of the Earth. However, even if you have access to the most advanced optical telescopes, the image you can get will look something like this:
')

Here is the raw image of the star GJ 758. It is made at a wavelength of 1.6 µm, so the colors here are false - a change in color from black to red shows only a change in brightness. Black spots in the center are areas so bright that it was not possible to accurately measure their value at the current settings of the equipment. Finally, white rays are diffraction distortions that appeared in this case due to the presence of so-called stretch marks in the telescope — structural elements that hold the telescope's secondary mirror.
Two candidates for the title of exoplanets rotate around GJ 758, however, it is not possible to distinguish them in this image, since a huge halo completely “clogs” their light. To detect these objects, it is necessary to “clear” the image from the light of the star itself as best as possible - but how to distinguish starlight from the light of the planet and diffraction distortions? In order to do this, you must first understand why distortions appear at all.
Distortions
The most obvious of the sources of distortion that form halos around a star is the atmosphere of the earth. Starlight, passing through it, is distorted even before it falls on the telescope mirror. Moreover, since the atmosphere is always in motion, these distortions are not constant, but changeable, and they change very quickly - every few tens of milliseconds. It is this atmosphere scattered by the atmosphere that will look like a soft halo around a star. Modern optics allows to reduce the number of such distortions to some extent, but cannot completely eliminate them.
Another major source of distortion is flaws in the telescope mirrors, as well as extensions on which the secondary mirror is attached. These distortions will be much more permanent - they will change, for example, due to temperature fluctuations, or due to movement of some parts of the telescope relative to others. Because of this constancy, such distortions are called pseudostatic. It is pseudostatic distortions that are responsible for the appearance of dark spots on the halo, which, although similar to planets, are in fact not.
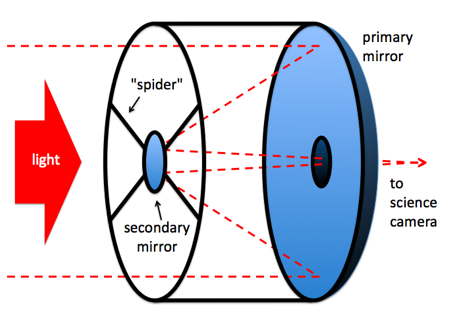
The scheme of the mutual arrangement of the primary and secondary mirrors of a large telescope.
Field rotation
Having dealt with the distortions, let us move on to the peculiarity of observations, which made the application of the ADI method possible.
Let's look at the night sky. Instead of an infinite cosmos, an observer from Earth could well imagine a huge sphere that surrounds our planet and onto which stars are projected. From the point of view of this observer, the celestial sphere will rotate slowly, making one full turn in about 24 hours. This is caused by the rotation of the Earth around the axis, and complicates the process of observation, since the stars, rotating with the celestial sphere, will strive to “escape” from the telescope's field of view. In order to compensate for this, telescopes are mounted on special rotating platforms that allow you to both adjust the inclination angle (measured from the horizon) and rotate the telescope to the desired azimuth. After aiming at the target, the telescope starts to rotate at a low speed, so that the visible image appears stationary. It is also worth noting that when the celestial sphere rotates, the mutual position of the stars relative to each other and the sphere itself remains unchanged. This whole process is shown in the following image:

Here the black squares with the stars are the field of view of the telescope — that portion of the sky that we are observing. As you can see, the field constantly rotates, but the relative position of the stars relative to each other and relative to the pole of the celestial sphere remains unchanged. If we want to constantly get a fixed picture, we will have to rotate the telescope at a certain angle, shown by the red arrows. This angle between the target, the zenith, and the pole of the celestial sphere, is called the parallax angle. It constantly changes during the night, but the speed of this change is not constant - it will be greatest when our target is at the zenith.
ADI technology
Using the fact that the field of the telescope rotates with respect to it during the observation, the ADI method allows us to distinguish the halo caused by distortions from the real light sources.
As we have already found out, with ordinary observations, the field of view, due to the rotation of the telescope, is kept at a constant angle to the observer. This allows you to take several pictures during the entire observation period and overlay them on each other. But when using the ADI method, the telescope is not rotated. At the same time, the true sources of light continue to rotate with the celestial sphere, but here the sources of pseudostatic distortion (for example, stretch marks) stop moving.
The following figure shows how a series of images taken by the ADI method are processed (they are designated as A
i ). First, you need to calculate the pixel-by-pixel mean between all the images obtained (B). On this average, those structures that did not change during the observation time (the very pseudostatic distortions, as well as the star itself) will be clearly visible, but the planet will hardly appear. Then, the obtained average is subtracted from each of the original images (C
i = A
i - B), with the result that only the planet and random small noises will remain. After that, each of the images will be rotated so as to correspond to the real coordinates of the celestial sphere (that is, the way they would have looked with the use of a derotator). Finally, from these rotated images D the mean E will be calculated again. On it all random noises will smooth out, but all the images of the planet will overlap each other, which will make them clearer.
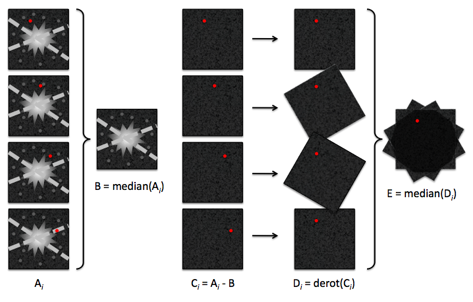
In the next image you will see the result of applying this method to the star from the very first image in this article. Despite the fact that the halo could not be completely removed (it was not completely static), ADI allowed us to isolate two objects located close enough to the star:

Object B in this image has been identified as a planet, and the status of object C has not yet been determined. White stripes above are shown to compare the size of the orbits of objects with the size of the orbits of some planets in the solar system.
It is worth noting that observations for using ADI should be done when the target is at its zenith, that is, when the field rotates at the highest speed.
LOCI
So, we have considered the easiest way to receive and process images - ADI. A more complex method, called Locally Optimized Combination of Images (LOCI), uses a rather convoluted algorithm that, instead of simply subtracting the average, makes it possible to better define the background on each of the images in the series. For this image are divided into ring segments, after which each of the segments is optimized separately. This allows you to better eliminate noise in images taken with a short period of time, and increases the contrast of the resulting image. In particular, the picture above was obtained using LOCI.
KDPV -
Telescope by Ryan Wick,
CC-BY