In the
first part of the review, I measured the laser parameters stated in the description and talked about the shortcomings in the design of the power supply. Now it was the turn to consider how the radiator itself is arranged.

To understand what is inside and why it is needed there, I would like to begin with a brief description of how lasers work at all. So:
Theory (boring)
A laser is an ingeniously simple device for understanding the principle of its functioning. At the same time, in order for the laser to work, you need to take into account a bunch of nuances, which opens up a huge scope for the work of engineers. It's like with an atomic bomb: here there are two pieces of uranium in half of the critical mass, we put them together - but no, something does not explode, only flows onto boots.
')
We all know that if an atom or molecule of a substance is given some energy, then after some time this atom / molecule will get rid of it - perhaps even by emitting a quantum of radiation (if it does not collide with any other atom before). This is spontaneous radiation, and so the light bulb works: the spiral is heated by electric current, the thermal energy of atoms (and tungsten and all impurities) is converted into radiation energy. In this case, the spectrum of such radiation roughly corresponds to the spectrum of an absolutely black body and is a bunch of different wavelengths with a characteristic peak of intensity for a given temperature.
At the same time, if an excited atom is hit by a photon of a certain frequency, without waiting for the atom to roll down to the lower energy level itself, then as a result of the absorption of such a photon, the atom reduces its energy to the photon energy and releases
two exactly identical photons that are identical to flew in Identical absolutely: in direction, in phase, in polarization, and, of course, in energy, i.e. wavelength. This is forced radiation.
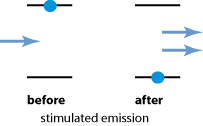
If we have many identical excited atoms, then the probability is high that a “doubled” photon will strike this atom, split again, and so on, until the excited atoms in the direction of wave propagation run out. Thus, just one photon of the correct wavelength, which has flown into space with our excited atoms, multiplies many times - it increases, and the atoms lose energy. From this it is clear that in order for the laser to work continuously, the emitting atoms continuously need to communicate the energy that takes them back to the upper energy level - “pump up”. Moreover, for successful amplification of atoms at the upper energy level should be greater than at the bottom, this state of matter is called the “inverse population”. One passage of an amplified quantum beam through the working medium is usually not enough, so it is placed in the resonator — two mirrors, one of which reflects the radiation completely, and the second partly releases the amplified beam.
The atoms discussed in the context of this laser are neodymium ions that are located at the lattice points of the yttrium vanadate crystal. If they just dangled in vacuum and were in the form of a gas, the laser would be gas, and since they are “fixed” in the crystal, the laser turns out to be solid-state. The crystal is chosen so that it is transparent for the wavelengths we need, strong mechanically, and is suitable for a number of other parameters that are not critical for understanding the work. Actually, a yttrium vanadate crystal of YVO
4 with an admixture (in other words, doping) with neodymium Nd is called the working body of the laser, and the entire formula is written as Nd: YVO
4 . Here it is important to understand that the main thing here is neodymium, and there are many crystals with suitable parameters for doping: Nd: Y
3 Al
5 O
12 (or shorter than Nd: YAG), Nd: YAlO
3 , etc. Everyone has some nuances but the essence is the same.
In the example of stimulated radiation, our atom had only two energy levels - upper and lower, but the reality looks more severe:
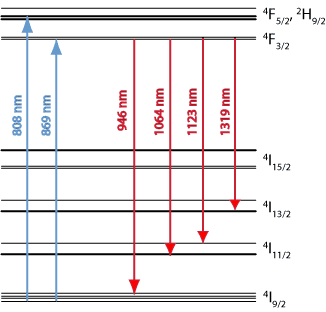
Here we see energy levels of a neodymium ion that are “interesting” in terms of emission and absorption in a yttrium-aluminum garnet crystal. It should be understood that a neodymium ion (like any quantum object) can absorb only quanta of certain wavelengths - the energy of which corresponds to the difference in energy of its levels. These are blue arrows.
Although it is energetically much more profitable to pump a crystal with a wavelength of 869nm, there are no powerful and cheap sources of such a wavelength. Therefore, laser diodes emitting 808nm (but intensively) are used, which drive ions to a level higher than necessary. After a short time, a nonradiative transition to the
4 F
3/2 level occurs. This is the so-called. metastable energy level. "Metastable" means that at this level the ion remains for a relatively long time, without dumping energy, but at the same time, this level is not the main one (not with the minimum energy). This is important, because in this state the neodymium ion must “wait” for its quantum, which will be enhanced with a transition to a lower level.
An excited neodymium ion can emit a quantum with one of four wavelengths suitable for further amplification (red arrows). Moreover, although the highest probability of radiation is at a wavelength of 1064nm, other transitions are also possible. They are struggling with them, using
dichroic mirrors of the resonator, which reflect only waves of length 1064nm, and the rest are released outside, preventing them from amplifying in the resonator. In this way, one or more of the possible laser radiation frequencies can be selected simply by replacing the mirrors.
So, pumping our crystal into a resonator with a laser diode, we receive laser radiation with a wavelength of 1064nm. It should be noted that neodymium can be pumped up not only with a laser diode, but also with flashlights and other radiation sources that have the necessary wavelengths in the spectrum, i.e. It is the laser as the source of the pump here is not required. Simply, a laser diode is very effective in terms of converting electrical energy into radiation of
one frequency we need (efficiency reaches more than 50%), and the fact that its radiation has polarization and coherence is a positive, but not mandatory quality.
The 1064nm infrared radiation turns into a green 532nm in a process called “second harmonic generation” (SHG). I'm afraid I will not be able to easily explain the essence of this process without doubling the volume of the article, so let's just assume that the nonlinear crystal in which this takes place is a black box that receives two quanta at the input, but only doubles the output frequency . Moreover, the efficiency of this process depends on the amplitude of the wave corresponding to the quantum (this is its nonlinearity), so looking through the crystal at the surrounding world, we will not see any color shifts - the light intensity is too small. But with laser energy densities, these effects appear in all their glory.
As with the working fluid, there are many non-linear crystals: KTP (potassium titanyl phosphate, KTiOPO
4 ), LBO (lithium triborate, LiB
3 O
5 ) and many others - all with their own pros and cons. In continuous (
CW ) lasers, a nonlinear crystal is placed inside the resonator in order to achieve greater polarization of the dielectric due to the repeated passage of the IR beam through the crystal and thereby increasing the efficiency of second harmonic generation. Lasers of this design are called intracavity second harmonic generation lasers. In pulsed lasers this does not bother - the energy density in the pulse is enough to complicate the resonator.
All DPSS lasers of average power are based on approximately one optical scheme:

LD is a pumping diode, F is a focusing lens,
HR is an input mirror (transmits 808nm and reflects 1064nm), Nd: Cr is a neodymium-doped crystal (a reflective coating for 532nm is deposited on its right-hand surface), KTP is a nonlinear crystal,
OC - output mirror (reflects 1064nm and passes everything else).
The HR and OC mirrors form a hemispherical Fabry-Perot resonator. The HR mirror is usually deposited on the crystal of the working medium; it is attempted to be made with the maximum reflectivity for the wavelength generated by the laser. The reflecting ability of the OC mirror is chosen so as to maximize the laser efficiency: the higher the gain of the medium (ie, the fewer passes through the crystal with neodymium the beam needs to be made enough to increase), the greater the transmittance.
As can be seen from the diagram, the only element that delays the radiation of 808nm from the laser diode is the crystal of the working medium. All that he could not absorb, passes through the mirrors in the exit aperture. Therefore, a dichroic filter is usually placed after the OC mirror, which reflects the unabsorbed pump radiation.
Now, knowing the basic theoretical principles of the laser and the basics of its design, we can proceed to the next part.
Practice
Unscrew the bottom panel and gain access to the four screws that secure the top cover:
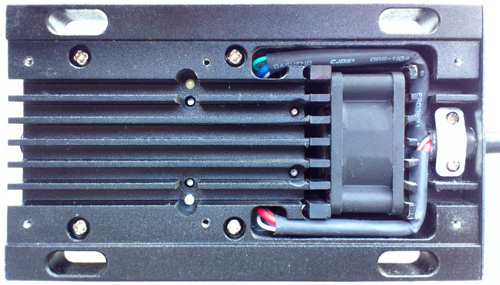
Carefully remove the cover, moving it forward so as not to hit the lens:
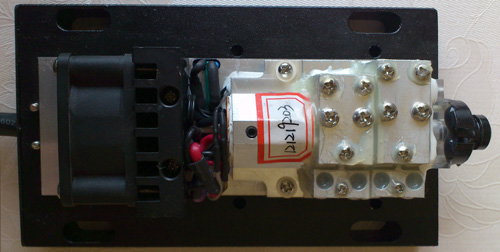
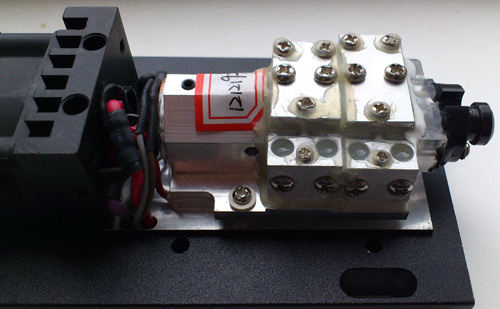
The laser itself occupies a relatively small volume of the radiator. One can see two adjustable optics holders - this is a good sign: it means, firstly, there is something to adjust, and secondly, it means that the laser is
not made on the "gluing" of the working fluid and a nonlinear crystal. Gluing unsuitable for extracting large capacity and can not be adjusted.
All slots are carefully smeared with silicone gel, which excludes access of dust and moisture to the resonator. A pair of adjustment screws located in the center on the top and to the side of each of the holders. The base of the laser is attached to the radiator with just two screws that press it to the thermoelement. Thus, the front edge of the platform just hangs over the radiator, which casts doubt on the overall rigidity of the structure.
There is no free space between the optical elements: my idea to put a mode aperture into the resonator and an IR filter in front of the lens was doomed to failure. Of course, frequency standards and other optical elements are out of the question; laser design does not imply modification.
Remove the fan to access the laser diode
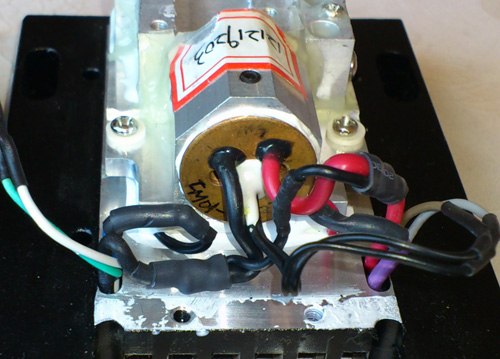
We remove the lens and both holders:
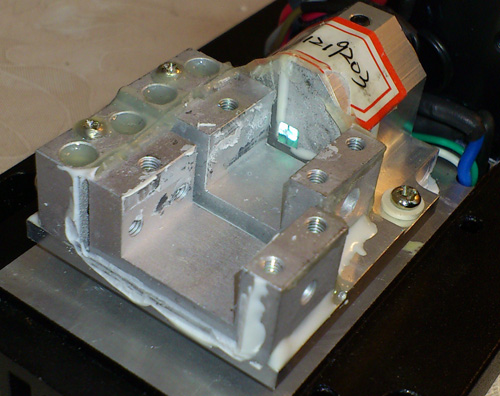
A view of a yttrium vanadate crystal 5x5x3 mm in size opens, which can withstand up to 15 W of pumping and output up to about 6 W of radiation at a wavelength of 1064nm. The proportion of neodymium impurity is most likely about 1 atomic percent. An antireflection coating for 1064nm and a reflective for 532nm are applied to this side.
Now look at the elements in the adjustable holders.
The holders are made of duralumin, allow adjustment in the horizontal plane with side screws and in the vertical one - with the upper screws. The adjustment is supposed to be performed as follows: loosen both screws for the same axis, then find the desired position of the holder with one of the screws and fix it with the second screw. Screws - the most common Chinese M3, not micrometric or accurate.
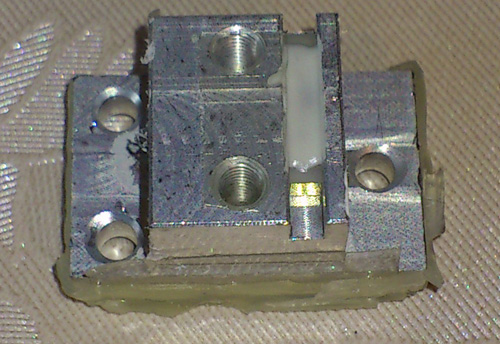
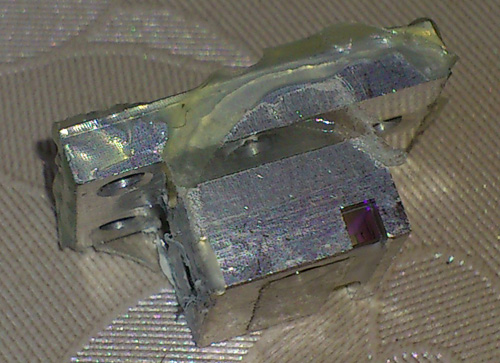
The KTP crystal is 3x3x7 mm in size, and in theory it can "serve" much more power - up to about 20 W @ 532nm. An antireflection coating is applied on its ends for waves of 532 and 1064nm length, the reflection coefficient of which is less than 0.5%. To align the crystal, it would be nice to have a third degree of freedom - rotation along the resonator axis, but then the manufacturers relied on the accuracy of cutting and gluing.
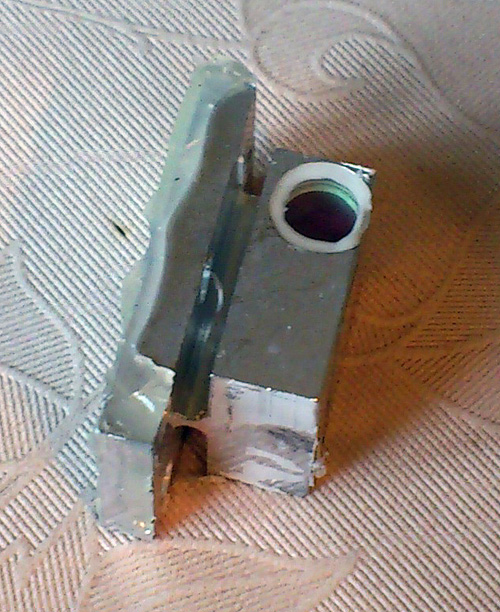
A dichroic concave mirror is glued into the output holder (no concavity is visible in the eye): it transmits light at a wavelength of 532nm and reflects 1064nm. At the same time, a significant part of the 808nm radiation also passes through it.
Remove the laser diode

The diode in the F-mount package is fixed on a massive brass base with thermal paste applied on it. In this type of case there is a hole for the installation of a thermistor that controls the temperature of the diode; The thermistor is present at a regular place. Produced diode company Focuslight; because except for the serial number, there is no other marking on it, its power is most likely 5 W - this is the lowest power for diodes in such a package, and it is logical to assume that the Chinese will not put anything more powerful and expensive there. Based on the
datasheet for this type of diode, the maximum current is 5.5A, i.e. Without exceeding the permissible values, the current set at the factory can be increased by 200 mA, which should add about 50 mW of output power. The diode can easily be replaced by a 10-watt one, the rest of the components allow, and I can’t get more than 3 W of a green beam at the output (I don’t dare to judge its quality, stability and mode composition).
The mounting of the diode allows you to rotate it along the axis of the resonator in order to choose the optimal polarization of the pumping radiation.
View of the working body from the side arc
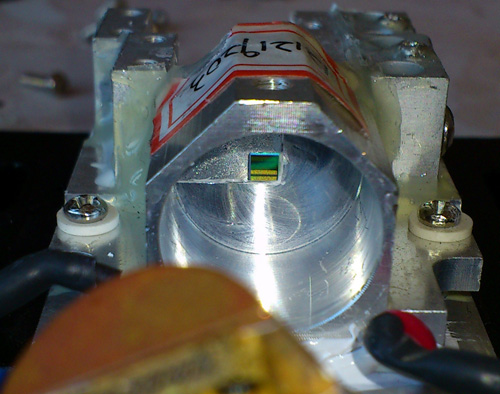
An antireflection coating for 808 nm and reflecting more than 99.5% for 1064 nm, which forms a flat mirror of the resonator, is deposited on this side of the crystal.
As we see, there is no focusing optics between the diode and the crystal: this reduces the pumping efficiency.
Turn off the base of the laser from the radiator
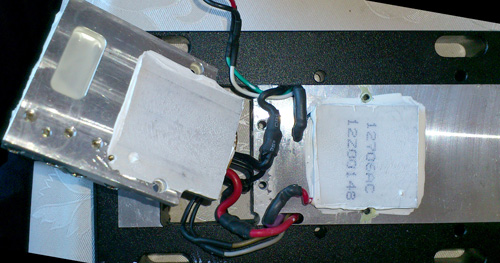
Under the base is the common Peltier element brand TEC1-12706. Its characteristics: power supply up to 15V, current up to 6A, output power up to 50W at a hot surface temperature of 60 ° C; dimensions 40x40x4 mm. A hole is made under the output optics holder - probably for a heating element with a different layout: a nonlinear crystal would be mounted in this holder, a focusing optics would be attached to the previous one, and the output mirror would be mounted separately (at the same time this would partially solve the problem with temperature expansion of the base). But this is just my guess.
Putting it all back
Needless to say that after the assembly the laser did not work? However, I quite quickly caught the generation, playing by adjusting the output mirror. Further adjustment of the mirror was not difficult. With the alignment of the KTP crystal, everything turned out to be much more difficult: to be honest, I can’t imagine how the Chinese did it by twisting the Philips screws with a screwdriver. Therefore, I replaced all the adjustment screws with hexagon bolts, which made it possible to perform a more precise adjustment with the key, while not pressing on the fasteners.
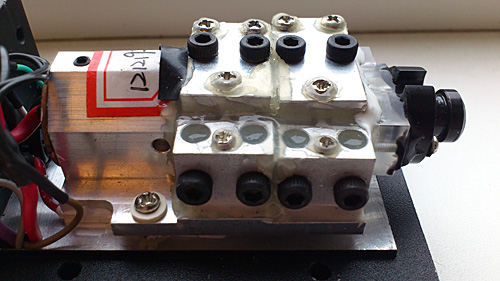
And even despite this, I did not succeed in fixing the exact critical angle of the KTP: all the same, the beam power noticeably jumps even with simple pressure with a finger and even by itself. Here it should be noted that the generation was in a very wide range of crystal alignment, but in some positions the power increased abruptly and also dropped abruptly with the slightest external disturbances. As a result, remembering the bike about the technician who used the pliers to punch the laser to replace the backlash mirror, I managed to achieve a stable power of about 1,650 mW, that is, the loss was about 200 mW.
Now it becomes clear why these lasers have such a large variation in power: it is possible that 1.8 W became possible only due to a happy impact during transportation, and from the factory the laser went out with a completely different power. Unfortunately, no laser test form was attached.
Conclusion
The crystals in the laser did not save: they allow much higher pumping power. I suppose that this is done for unification, and the three-watt laser differs from the one-watt laser only by the power of the laser diode, the power supply and three times the price. The rigidity and accuracy of the mechanics leave much to be desired - you can see the desire to make inexpensive, but at least the design is maintainable. The declared durability of the design seems to be determined mainly by the durability of the laser diode (and it was not possible to find it in the documentation) and the cleanliness of the assembly room — I did not see any impurities in optics when disassembling the laser.
And summing up, I want to answer the main question to the first part of the article, which arose among many - “Why does this laser need such a laser at all?” Proceeding from its power, which is insufficient for efficiently pumping titanium sapphire and dyes, mode composition and stability, which also so-so, the main scope of its application - OEM component for laser projectors. It can also be used for illumination: for recording luminescence, in confocal microscopy, etc. areas where high backlight power is required at a relatively stable frequency.