Recently, I discovered a very sad fact: on Habré such a funny topic as DNA origami is not covered at all. There is only
one post in 2009 , telling only the very beginning of an entertaining story about how from DNA (yes, that
deoxyribonucleic acid carrying our genetic information) you can create all sorts of tricky, flat and three-dimensional pieces of nanometer size. That same nano-technology as it is. In this review, I want to talk about the development of DNA origami: two-dimensional DNA smileys, three-dimensional shapes, crystals of DNA with a programmed structure, DNA “boxes” with a lid that can carry the necessary substances and release them after the signal about opening the lid, and Finally, dynamic structures like a DNA walker walking on a substrate (the creators proudly say that this is already a nanobot!). Who wants to learn more about why all this is necessary, read about the technology of making beautiful nanometer pieces from DNA, or just to see beautiful pictures, welcome under cat.
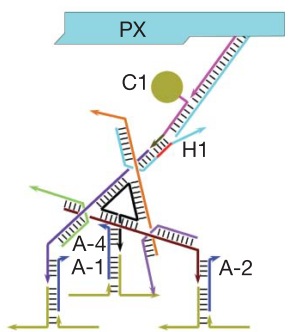
This is the DNA nanobot
')
Some theory
At the end of the twentieth - the beginning of the twenty-first century, the question arose of constructing nanometer-sized objects. For what? The common vector for miniaturization has been around for a long time, and historically it has always been a “top down” movement - for example, in the 70s, when manufacturing microchips, the minimum controlled size was 2-8 microns, then this value was rapidly decreasing and now there are chips in mass production , made by 22-nm process technology. Then the thinking people had a question: is it possible to move “from the bottom up”? Is it possible to make atoms and molecules assemble into the necessary structures and then use these structures in a technique? The requirements for such a “self-assembling” system are obvious: the materials for it must be fairly cheap and accessible, the self-assembly of the complex spatial structure of the system must be easily and obviously “programmed”, the system must be capable of carrying useful functionality. They immediately recalled that in nature such self-assembling systems already exist and work fine - these are macromolecules of all living organisms, for example, proteins. Here comes the first disappointment - proteins are too complex, their three-dimensional structure is given in a completely unobvious way by many non-covalent interactions and getting a protein with an arbitrary structure is still an absolutely non-trivial and unsolvable task. That is, it is technically impossible to use proteins for constructing the required nano-sized objects. What to do? It turns out that there are other macromolecules whose structure is much simpler than the structure of proteins.
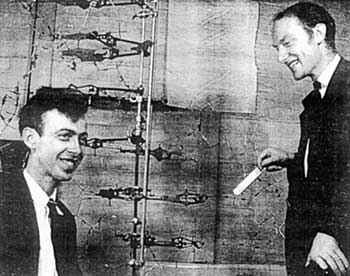
In 1953, Watson and Creek published their
DNA structure model , which turned out to be absolutely correct. DNA (deoxyribonucleic acid) is an interestingly arranged linear polymer. One DNA strand consists of a monotonously repeating sugar-phosphate backbone (it is asymmetric and has a direction, there are 5 'and 3' ends of the chain), but each of the sugars (deoxyribose in the case of DNA) is attached to one of four nucleotides (the synonym for the word nucleotide - “base ") - adenine, or thymine, or cytosine, or guanine. Usually they are denoted by one letter - A, T, C, G. Thus, DNA contains only 4 types of monomers, unlike 20 amino acids in the protein, which makes the structure of DNA much easier. Then it becomes even more fun - there is the so-called “Watson-Cricova base pairing”: adenine can specifically bind to thymine, and guanine - to cytosine, forming pairs - and - (and also - and -, of course ), other interactions between nucleotides in the simplified case can be considered impossible (they are possible as an exception under some rare conditions, but for us this is not important). Watson-Cricova base pairing is also called complementarity.

Two DNA chains, the base sequence of which is complementary, immediately “stick together” into a double helix. The question arises: what if there are two complementary regions on one DNA chain? Answer: the DNA chain can bend and the complementary areas can form a double helix, and together with the bend, this structure will be called the “hairpin” (DNA hairpin):

What is the basis for the "sticking together" of two complementary strands of DNA (or, similarly, of two complementary portions of one strand)? This interaction is based on
hydrogen bonds . The A – T pair is connected by two hydrogen bonds, the G – C pair is three, so this pair is more energy stable. About hydrogen bonds, one should understand the following: the energy of one hydrogen bond (5 kcal / mol) is not much higher than the energy of thermal motion, which means that a single hydrogen bond can be destroyed with a high probability by thermal motion. However, the more hydrogen bonds, the more stable the system becomes. This means that short sections of the complementary bases of DNA cannot form a stable double helix, it will easily “melt”, however longer complementary areas can already form stable structures. The stability of the double-stranded structure is expressed by one parameter - the melting point (Tm, melting temperature). By definition, the melting point is the temperature at which in equilibrium 50% of DNA molecules with a given length and sequence of nucleotides are in the double-stranded state, and the other 50% in the melted single-stranded state. Obviously, the melting point directly depends on the length of the complementary region (the longer - the higher the melting point) and on the nucleotide composition (as there are three hydrogen bonds in the D-C pair, and two in the A-T pair, -C, the higher the melting point). The melting point for a given DNA sequence is easily considered according to an
empirically derived formula .
From theory to practice
So, we studied the theory. What can we do in practice? With the help of chemical synthesis, we can directly synthesize DNA strands up to 120 nucleotides in length (then the output of the product just drops sharply). If we need a longer chain, then it can easily be assembled from the very chemically synthesized fragments up to 120 nucleotides in length (for example, Uncle
Craig Venter distinguished himself by
collecting 1.08 million base pairs of DNA from the pieces). That is, in the 21st century, we can easily and cheaply make DNA of any sequence that we want. And we want the DNA to fold into all sly and complex structures that we can use later. To do this, we have the principle of complementarity - as soon as complementary zones appear in the DNA sequence, they stick together and form a double-stranded region. Obviously, we want to make structures that are stable at room temperature, which means we want to calculate the melting point for these areas and make it rather large. At the same time, we can make many different regions with different sequences on the same DNA strand and only complementary ones will stick together. Since there can be several complementary regions, as a result, the molecule can collapse in a rather complicated way! Somehow, for example:

Also, in addition to the positive design (creating areas that can form the structure we need), when developing structures with self-assembly, we should not forget about the negative design - we need to check the resulting DNA sequence for the potential presence of parasitic interactions (when parts of the areas we created are able to interact to another, forming parasitic structures unnecessary for us) and get rid of these parasitic structures and interactions, changing the nucleotide sequence of DNA. How to get the simplest DNA structures of the "hairpin" type is quite obvious, but boring and uninteresting. Is it possible to make something more difficult from DNA? Here, no computer calculations can not do. We want a certain structure and now have to pick up the DNA sequence, which will collapse into this structure due to the interaction of complementary regions, but there should be no parasitic interactions, unintended complementary regions that form alternative structures in the sequence. Plus, the structure must meet other criteria, for example, have a melting point above a certain specified value. As a result, we have a typical optimization problem.
Two-dimensional DNA structures
Methodological breakthrough gave Paul Rothemund (California Institute of Technology) in 2006, it was he who coined the term "DNA origami". In his
article in Nature, he presented a variety of fun two-dimensional objects made from DNA. The principle proposed by him is quite simple: take a long (approximately 7000 nucleotides) “supporting” single-stranded DNA molecule and then, using hundreds of short DNA clips that form double-stranded regions with the supporting molecule, bend the supporting DNA into the two-dimensional structure we need. Here is a drawing from the original article, representing all the stages of development. To begin with (a) let's draw the shape we need in red and figure out how to fill it with DNA (imagine it at this stage in the form of tubes). Further (b) we will present how to carry out one long basic molecule on the form necessary to us (it is shown by the black line). At the third stage (c) we will think about where we want to place the “clips” that stabilize the laying of the long supporting chain. Fourth stage (d): more details, we figure out how all the DNA structure we need will look and, finally, (e) we have the scheme of the structure we need, you can order the DNA of the desired sequence!
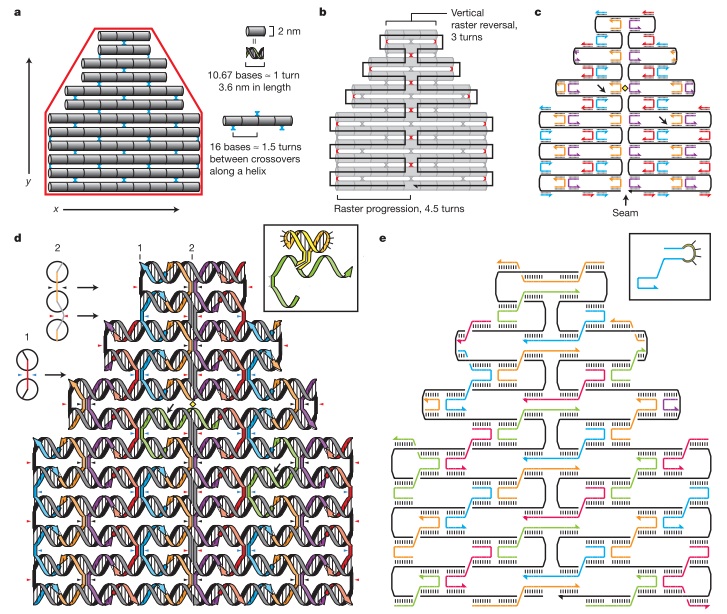
How can we assemble the structure we need from chemically synthesized DNA? Here comes the process of melting. We take a tube with an aqueous solution, throw all the DNA fragments into it and heat it to 94-98 ° C, the temperature that surely melts all the DNA (translates it into single-stranded form). Then we just very slowly (for many hours, in some works - for several days) cool the tube to room temperature (this procedure is called annealing, annealing). At this slow cooling, when the temperature is sufficiently low, double-stranded structures that we need are gradually formed. In the original work in each experiment, about 70% of the molecules were successfully collected in the desired structure, the rest had defects.
Further, after the structure is calculated, it would be nice to prove that it is going exactly as we need. For this, atomic force microscopy is most often used, which just perfectly shows the general shape of the molecules, but sometimes cryo-EM (electron microscopy) is also used. The author made a lot of fun forms from DNA, the pictures show the calculated structures and the result of the experimental determination of structures using atomic force microscopy. Enjoy!
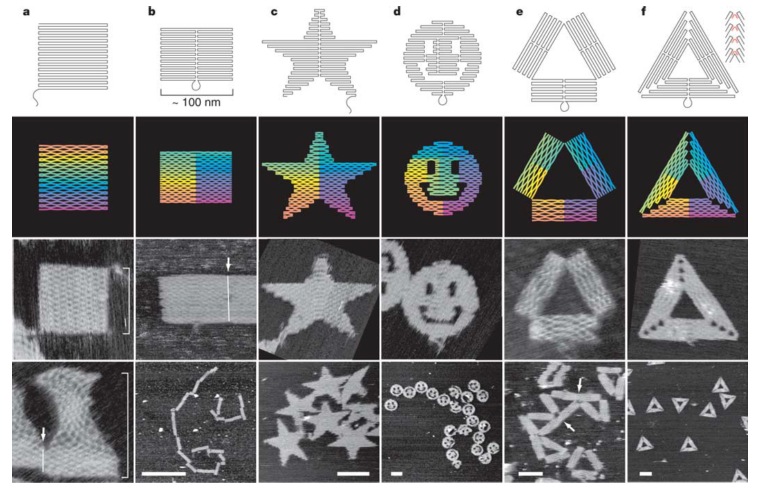
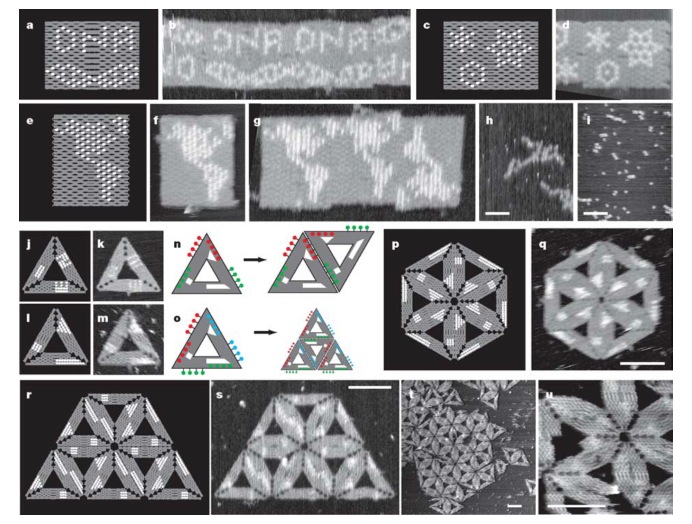
Three-dimensional DNA structures
After you have dealt with the design of complex flat objects, why not move on to the third dimension? Here, the pioneers were a group of guys from the Scripps Institute in La Hall, California, who in 2004 came up with how
to make a nano-octahedron from
DNA . Although this work was done 2 years earlier than flat DNA origami, at that time only a special case was solved (obtaining an octahedron from DNA), but in the work on DNA origami, a general solution was proposed, therefore it was the 2006 work on DNA origami considered fundamental.
The octahedron was made from a single-stranded DNA molecule of about 1,700 nucleotides in length, which has complementary regions and is also linked by five 40-nucleotide DNA adapters, resulting in an octahedron with a diameter of 22 nanometers.
In the figure, note the color coding on the two-dimensional scan of the octahedron. See areas marked with the same color? They contain both complementary zones (parallel sections connected by cross links) and non-complementary (in the diagram they are depicted as bubbles), while zones of the same color, located in different parts of the two-dimensional sweep, interact with each other, forming a complex structure depicted in Figure 1c and forming the face of a three-dimensional tetrahedron. Enjoy beautiful pictures!
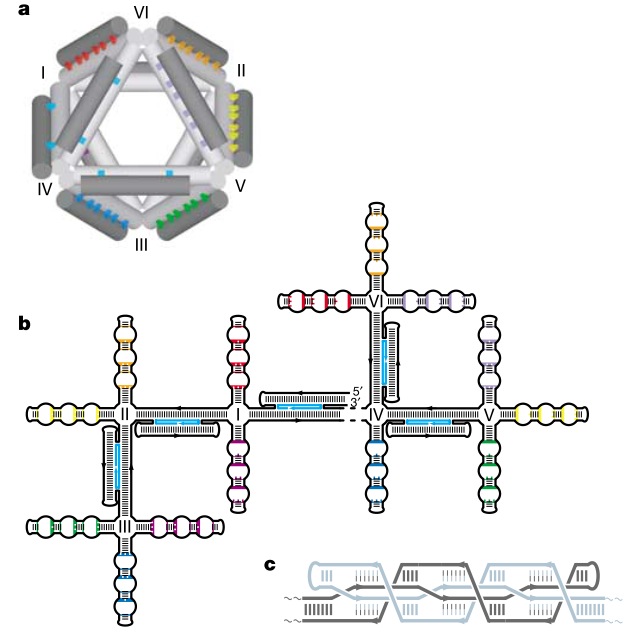

In 2009, scientists from Boston and Harvard University published the principles of constructing
three-dimensional DNA origami , as they say, in the likeness of a honeycomb. One of the achievements of this work is that people wrote
the caDNAno open-source program for constructing three-dimensional DNA structures (it works for Autodesk Maya). With this program, even a non-expert can assemble the necessary structure from ready-made blocks using a simple graphical interface, and the program will calculate the necessary DNA sequence (or sequences) into this structure that collapses.

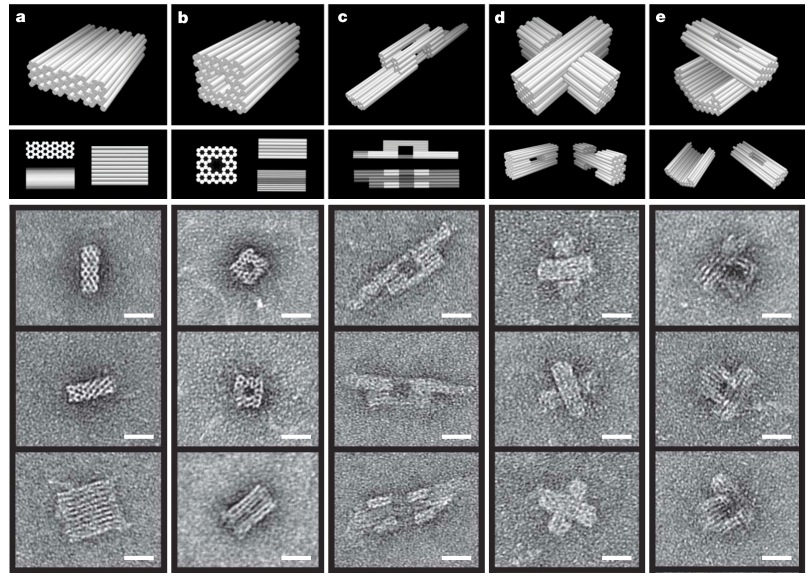

In
their next work, they learned how to make complex three-dimensional objects with controlled curvature from DNA and pleased the readers of Science magazine with beautiful pictures of different curved objects from DNA (there such cool gears turned out!).



Periodic structures

Until now, scientists have played with non-periodic structures of DNA. And what if you make such a structure so that one block can interact with another such block, and so on ad infinitum? Imagine three pieces at a 90 degree angle to each other (like an anti-hedgehog). It is obvious that such a structure can be a node of an infinite cubic lattice, if each side of such a hedgehog interacts with another such hedgehog. It was this idea that scientists from New York
put into practice in 2010, they made such a DNA hedgehog, which immediately formed a three-dimensional lattice, that is, a crystal from DNA, so they used X-ray analysis to show that DNA formed exactly this structure what they wanted. In turn, since the DNA crystals had a size of up to half a millimeter (and this is already a macro object), it was proudly stated that now we can assemble macro objects from nano-objects.

Here is a stereo picture of the lattice site, if you know how to mow your eyes correctly (this is a direct stereo pair), you can see it in 3D (in the bottom picture with DNA density, two “anti-tank hedgehogs” are clearly visible):

Dynamic structures
The next step in the design of three-dimensional nano-objects is also obvious - but what if you make it all move somehow? The moving object can already be called a nano-robot. The simplest DNA walker was made in 2008 by a
team from the California Institute of Technology . It works on a fairly simple principle. Imagine single-stranded DNA with a length of, say, 100 nucleotides. Imagine another single-stranded DNA, short, 50 nucleotides long, complementary to half of the first molecule. What happens if they mix? True, they form a double-stranded structure in the region of the 50 nucleotides, while the second half of the first molecule will remain free. And what if to this structure add another DNA molecule, 100 nucleotides in length and completely complementary to the first molecule? The answer is quite obvious: it will displace a short chain of 50 nucleotides in length, since it has a high affinity for the first molecule (they have an affinity of 100 out of 100, and a short one has only 50 out of 100 nucleotides). That is how the first DNA walker worked. Single-stranded DNA molecules are fixed on the substrate; a walker molecule comes to them from the solution, which has complementary zones to two adjacent chains on the substrate and binds to them. If then we add to the solution another DNA that has a greater affinity for the first strand on the substrate, then it will displace one leg of the walker, after which this leg will contact the next (third) strand of DNA on the substrate. By adding new replacement DNA, you can drive the walker farther and farther along the substrate. Reverse is impossible, since the previous chains on the substrate are already inactivated by binding to a longer DNA molecule.
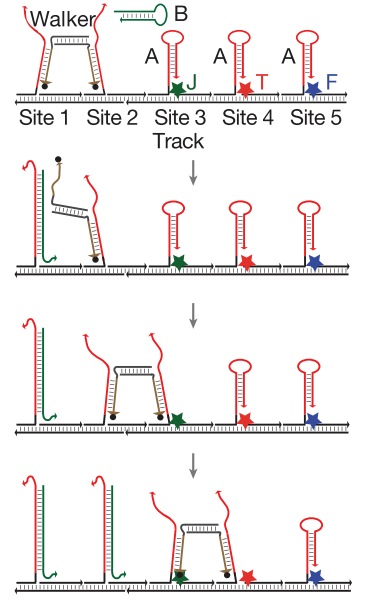
Although the first walker looked so primitive, the design was
refined by scientists from New York . They made a more complicated walker with several “hands” and “legs”, hooked the “weight” (gold particles with a diameter of 5 and 10 nm) onto the substrate using “complementary” DNA and “programmed” the walker so that it went over the substrate and collected the load - three small and one large gold particles. The sequence of steps is easy to track down the arrows, and in the experimental picture on the right you can see gold particles and how the walker collects them. Nano-robot in action! The bottom picture shows exactly how the process of “stepping” and collecting the load takes place, the principle is the same, the crowding out of one DNA by another.


But that is not all. The crown of DNA robotics (I think we hear some sarcasm in my voice) was
“a nanobot for molecular transport,” as the creators from Boston dubbed it. In fact, the guys made some kind of a “box” of DNA that closes with a “lock” of DNA, which can be opened according to the principle we already know of displacing one DNA by another, as in walkers. A cargo is hidden inside the box - particles of gold or immunoglobulin molecules. DNA can be chemically modified so that it can be fixed to this very load. So, we have a closed box, the contents of the box are safely hidden. Here we add the molecule that opens the box, it opens and the immunoglobulin, hidden inside, goes outside and begins to act! We clap our hands, we love and we rejoice at the progress.

The guys were not even too lazy to make a demonstration of proof of principle on living cancer cells: they hid antibodies in the box that blocked the key proteins of the cell cycle, injected the boxes into the cancer cells, and after adding the activator that opens the box, the cancer cells really stopped to divide! Thus, it was shown that it is possible in principle to use such structures for targeted drug delivery in the body and their release at the right time by a signal from an activator molecule. One problem remains, how to reliably distinguish a healthy cancer cell ...
Why goat bayan?
All this, of course, is healthy and good, the pictures are beautiful, but an attentive reader may ask: “Where are the benefits for the national economy promised at the very beginning?”. As with any new technology, there is really no great practical benefit, apart from the aesthetic pleasure from the contemplation of this nano-beauty. However, everything is just beginning! First, DNA can be chemically modified, chemical groups can be added to it, which can bind to other molecules, and then DNA can be used as a substrate to build complex structures from other molecules. For example, now everyone wants to make something from nanotubes. If it is possible to make an adapter that binds one end to DNA and the other to a nanotube, then DNA structures can be used to connect nanotubes. On the other hand, there are already
reports of controlled DNA metallization , and from here it is already within reach to construct electronic devices based on structures from metallized DNA. Perhaps scientists will invent a more suitable polymer that provides a more convenient self-assembly of complex structures, but in any case, DNA origami will take its place in the history of science as one of the first examples of designing complex objects on a nano-scale. Anyway, a great and bright future awaits us, which is what you want!
PS: an interesting addition from
vxsw :
“For a couple of years, a
BIOMOD competition has been held for the design of such pieces with the support of the
Wyss Institute (seen in many interesting biotechnological achievements, such as the recent
3D printing of mini-batteries ).”
PPS: in a personal, asked why DNA, not RNA. The answer is this: I see two main reasons: (1) DNA is chemically more stable. All living organisms synthesize huge amounts of RNases, enzymes that destroy RNA. If you accidentally climb into the tube with RNA with your bare finger, there will be nothing left of RNA - everyone will eat RNases. Therefore, they work with RNA in special rooms and so on - the troubles are much more than when working with DNA. There are no such problems with DNA, you put your finger in the test tube - there will be nothing DNA. (2) The cost of chemical synthesis of RNA is several times higher than the cost of DNA synthesis. I think that's why people have fun with DNA - cheaper and easier.