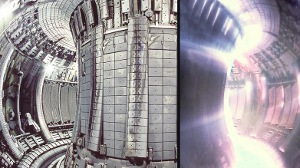
As a child I loved to read the magazine "Science and Life", in the village lay filing since the 60s. There they often talked about thermonuclear fusion in a joyful way — almost now, and it will be! Many countries built Tokamaks in order to have time to distribute free energy (and set up a total of 300 pieces around the world).
The years went by ... Now is the 2013th year, and humanity still receives most of the energy from burning coal, as in the 19th century. Why it happened that prevents to create a thermonuclear reactor, and what can we expect in the future - under the cut.
Theory
The nucleus of the atom, as we remember, consists in the first approximation of protons and neutrons (= nucleons). In order to detach all neutrons and protons from an atom, it is necessary to expend a certain energy - the binding energy of the nucleus. This energy is different for different isotopes, and naturally, during nuclear reactions, the energy balance must be maintained. If we plot the binding energy for all isotopes (based on 1 nucleon), we obtain the following:
From here we see that we can receive energy either by separating heavy atoms (like
235 U), or by connecting light ones.
')
The most realistic and interesting in practical terms the following synthesis reaction:
1)
2 D +
3 T ->
4 He (3.5 MeV) + n (14.1 MeV)
2)
2 D +
2 D ->
3 T (1.01 MeV) + p (3.02 MeV) 50%
2 D +
2 D ->
3 He (0.82 MeV) + n (2.45 MeV) 50%
3)
2 D +
3 He ->
4 He (3.6 MeV) + p (14.7 MeV)
4) p +
11 B -> 3
4 He + 8.7 MeV
Deuterium (D) is used in these reactions - it can be obtained directly from seawater, Tritium (T) is a radioactive isotope of hydrogen, now it is obtained as a waste in conventional nuclear reactors, and it can be specially produced from lithium. Helium-3 - it seems to be on the moon, as we all already know. Boron-11 - natural boron consists of 80% of boron-11. p (Protium, hydrogen atom) - ordinary hydrogen.
For comparison, when dividing
235 U, ~ 202.5 MeV of energy is released, i.e. much more than the fusion reaction at the rate of 1 atom (but based on a kilogram of fuel - of course, thermonuclear fuel gives more energy).
By reactions 1 and 2, a lot of very high-energy neutrons are obtained, which make the whole reactor construction radioactive. But the reactions 3 and 4 - "non-neutron" (aneutronic) - do not give induced radiation. Unfortunately, side reactions still remain, for example, from the 3-D reaction, deuterium will react with itself, and there will still be some neutron radiation.
Reaction 4 is interesting because as a result we get 3 alpha particles, from which theoretically it is possible to directly remove energy (since they are actually moving charges = current).
In general, there are enough interesting reactions. The only question is how easy it is to implement them in reality?
About the complexity of the reaction, Humanity has relatively easily mastered the division of
235 U: there is no difficulty here - since neutrons do not have a charge, they can literally “creep” through the nucleus even at very low speeds. In the majority of fission reactors, precisely such thermal neutrons are used - in which the speed of motion is comparable with the speed of the thermal motion of atoms.
But in the synthesis reaction - we have 2 nuclei with a charge, and they repel each other. In order to bring them closer to the right distance for the reaction, they need to move with sufficient speed. Such speeds can either be achieved in an accelerator (when all atoms as a result move with one optimal speed), or by heating (when atoms fly as they hit in random directions and random speed).
Here is a graph showing the reaction rate (cross section) versus the speed (= energy) of the colliding atoms:
Here is the same, but built from the temperature of the plasma, taking into account the fact that the atoms fly there at a random speed:
We immediately see that the D + T reaction is the “lightest” (it needs a measly 100 million degrees), D + D is about 100 times slower at the same temperatures, D +
3 He goes faster than competing D + D only at temperatures of the order 1 billion degrees.
Thus, only the D + T reaction is at least remotely accessible to man, with all its flaws (the radioactivity of tritium, the difficulty in obtaining it, the radiation induced by neutrons).
But as you understand, take and heat something up to one hundred million degrees and leave to react does not come out - any heated objects emit light, and thus cool down quickly. Plasma heated to hundreds of millions of degrees shines in the X-ray range, and the saddest thing is that it is transparent to it. Those. a plasma with such a temperature cools down fatally quickly, and in order to maintain the temperature, it is necessary to constantly pump in giant energy to maintain the temperature.
However, due to the fact that there is very little gas in a fusion reactor (for example, in ITER - only half a gram), everything does not work so badly: to heat 0.5 g of hydrogen to 100 million degrees you need to spend about as much energy as for heating 186 liters of water at 100 degrees.
There is another
Lawson criterion that shows whether a reaction will produce more energy than it spends. In addition to temperature, density is also important (of course, plasma density is higher - the reaction proceeds faster) and plasma retention time (in order to react). Accordingly, the systems can be pulsed (Z-Machine, NIF, thermonuclear charge - short reaction time, high temperature and density) and constant (tokamak - low density and temperature, long reaction time).
Let us now see what approaches there are to the implementation of a fusion reactor.
Constructions
The star is a natural thermonuclear reactor. Hot plasma under high pressure is held by gravity, and all the emitted x-rays are absorbed due to the enormous density and size. Thus, the core does not cool down even at relatively low reaction rates. Because of this, not only hydrogen and deuterium burn in the core, but also
much heavier elements . Unfortunately, on earth such a construction is difficult to implement.
Thermonuclear (hydrogen) bomb is also quite simple in design. A hollow plutonium sphere in the delta phase (the delta phase is 1/4 less dense than the alpha phase), and in the center in the simplest case is thermonuclear fuel, lithium-6 deuteride. With the help of 2 types of explosives (“slow” and “fast”) and two detonators, a spherical shock wave is formed, which converts plutonium into a smaller alpha phase, in which a fission chain reaction is possible. Optionally, you can add an external pulsed neutron initiator (below) - at the time of greatest compression, it will produce a bunch of neutrons, which should give a sharp start to the reaction.
The "extra" neutrons are captured by lithium-6 with the formation of tritium, and a heated mixture of deuterium and tritium is just needed. They begin to react with each other — and the inertia force of a relatively heavy charge body from uranium keeps them from flying away. In addition, the uranium body is opaque to x-rays - correspondingly less heat loss. The entire reaction ends in 1 microsecond - and the body is just starting to fly apart.
This was the so-called “booster circuit” of a nuclear charge, where the contribution of the thermonuclear reaction is small, and only allows a little to increase the power “cheaply” (plutonium is terribly expensive, and lithium is cheap as dirt in comparison with it).
Tritium is not directly used because it is radioactive and therefore not stored for a long time. And lithium-6 is stable, and the nuclear charge is always ready for battle. Lithium-7 can also be used - it not only gives tritium, but also another extra neutron. This reaction was not known when the Americans tested the Shrimp (Shrimp) bomb. Due to the lack of pure lithium-6, partially enriched in which lithium-6 was only 40% was put in, and they were counting on an explosion of 6 megatons, and hit 15.
There is also a radiation implosion scheme - when a primary nuclear explosion with X-rays compresses and heats a separate sphere with thermonuclear fuel.
Of course, this all works well for the purpose of destruction, but this approach cannot be used for energy, the minimum power of the explosion is too high, and there are too many ordinary radioactive plutonium / uranium reaction products.
Linear accelerators : the idea is simple - we take a target from any convenient metal deuteride, and in a small linear accelerator we accelerate tritium atoms to the desired speed. We get a real thermonuclear reaction, and an energy output and 14.1 MeV neutrons. Such a source can be used to search for oil and water (for example, on the Martian rover MSL there is a Russian pulsed neutron source DAN), and as an external pulsed neutron initiator in nuclear charges.
Why is it so impossible to generate electricity? A lot more energy is spent on the acceleration of atoms than we receive as a result of the reaction (not all of the atoms being accelerated react). According to
my calculations, for example, DAN has an efficiency of the order of 0.0016%.
Tokamak (toroidal camera with magnetic coils) - the idea is already a bit more complicated, in a plasma torus as in a transformer we induce a current. Around the torus are superconducting magnets that “compress” the plasma and prevent it from touching the walls. The plasma is heated by microwave radiation, and resistive heating from the flowing current. When they began to work in this area, it seemed: just about everything would work.
About 300 tokamaks have been built around the world, and the most modern and largest of them is the international
project ITER under construction (including with the participation of Russia). In it, the indicator Q = 10 should finally be reached (i.e., the energy release is 10 times more than that spent on heating and confining the plasma). Hydrogen plasma (i.e., without thermonuclear reaction) is going to be ignited in 2020, and to start launches with deuterium-tritium plasma - in 2027, of course, if everything goes according to plan and some new crisis does not happen.
The problems with tokamaks are the following (with their future industrial use):
- Plasma instability. The discharge strives somewhere becomes thinner, somewhere - thicker, until the ring breaks (with the cessation of current) or by touching the walls. They struggled with the problem of increasing the size of the camera, adding a poloidal magnetic field (around the vertical axis of the camera).
- Tritium is expensive and needs a lot to produce energy. If we are the only neutron produced in the D + T reaction with lithium-6 we convert tritium into 1 atom - due to the inevitable loss of neutrons, tritium will be less and less. It is necessary to use the multiplication of neutrons - using for example lithium-7 or lead, which you need to cover the inner wall of the reactor (blanket), and get some tritium out of there.
- Powerful neutron radiation: for the same output power, the neutron flux is ~ 5-10 times greater than that of conventional nuclear reactors, and the neutrons themselves have much more energy. This means that if the design of the reactor is made of the same materials, then its service life will be 5 years, not 50 (as in conventional reactors).
- Since the plasma with a huge temperature loses a lot of energy for radiation, and the chamber must be large to ensure stability - the minimum power of the reactor is large, hundreds of megawatts.
The stellarator is a “crumpled” bagel, where the magnetic field is formed by external magnets of a very cunning form and ensures the stability of the plasma. Compared to tokamak - a much more complex structure. The “quality” of plasma confinement is now inferior to tokamaks.
NIF - National Ignition Facility - the idea is to focus light from 192 pulsed lasers on a target surrounding a deuterium-tritium capsule. The light heats up the target - it heats up to millions of degrees, and evenly “compresses” the capsule with thermonuclear fuel. By the way, 3 years ago, they wrote on Habré that
almost everything was ready there .
The project ended on September 30, 2012. It turned out the computer model had inaccuracies. According to the new estimate, the pulse power of 1.8 megajoules achieved in NIF is 33-50% of the required energy so that the same energy is released as was spent.
Sandy Z-machine The idea is this: take a large pile of high-voltage capacitors, and sharply discharge them through thin tungsten wires in the center of the machine. The wires instantly evaporate, a huge current of 27 million amperes continues to flow through them for 95 nanoseconds. The plasma, heated to millions and billions (!) Of degrees, emits X-rays, and compresses them with a capsule with a deuterium-tritium mixture in the center (the X-ray pulse energy is 2.7 megajoules).
It is planned to upgrade the system using the Russian power plant (Linear Transformer Driver - LTD). In 2013, the first tests are expected, in which the energy received is compared with the expenditure (Q = 1). Perhaps in this direction in the future there will be a chance to compare and exceed tokamaks.
Dense Plasma Focus - DPF - “collapses” plasma running along the electrodes to produce gigantic temperatures. In March 2012, a plant operating according to this principle reached a temperature of 1.8 billion degrees.
Levitated Dipole is an
“inverted” tokamak , in the center of the vacuum chamber a toroidal superconducting magnet hangs and holds the plasma. In such a scheme, the plasma promises to be stable on its own. But now the project does not have funding, it seems that the synthesis reaction was not directly carried out at the facility.
Farnsworth – Hirsch fusor The idea is simple - we place two spherical grids in a vacuum chamber filled with deuterium, or a deuterium-tritium mixture, apply a potential of 50–200 thousand volts between them. In an electric field, atoms begin to fly around the center of the chamber, sometimes colliding with each other.
There is an output of neutrons, but it is rather small. Large losses of energy for bremsstrahlung X-rays, the internal grid quickly heats up and evaporates from collisions with atoms and electrons. Although the design is interesting from an academic point of view (any student can collect it), the efficiency of neutron generation is much lower than linear accelerators.
Polywell is a good reminder that not all thermonuclear fusion work is public. The work was funded by the US Navy, and was classified until negative results were obtained.
The idea is the development of Farnsworth – Hirsch fusor. The central negative electrode with which we had the most problems, we replace with a cloud of electrons held by a magnetic field in the center of the chamber. All test models had ordinary, not superconducting magnets. The reaction yielded single neutrons. In general, no revolution. Perhaps the increase in size and superconducting magnets would change something.
Muon catalysis is a radically different idea. We take a negatively charged muon, and replace it with an electron in an atom. Since a muon is 207 times heavier than an electron, in a hydrogen molecule, 2 atoms will be much closer to each other, and a synthesis reaction will occur. The only problem is if helium is formed as a result of the reaction (~ 1% chance), and the muon flies away with it - it will not be able to participate in the reactions more (since helium does not form a chemical compound with hydrogen).
The problem here is that muon generation at the moment requires more energy than can be obtained in a chain of reactions, and thus, until energy is obtained here.
“Cold” thermonuclear fusion (“cold” muonic catalysis is not included here) - has long been a pasture of pseudo-scientists. There are no scientifically confirmed and independently repeatable positive results. And the sensations at the level of the yellow press were already more than once before E-Cat Andrea Rossi.
Summary
- Thermonuclear energy is not so crystal clear at all. At the only realistic D + T reaction at the moment, the neutron flux that will make any structural elements radioactive is 10 times higher than in conventional reactors of the same power. The reactor vessel will have to be changed every 5-10 years.
- Mankind will certainly reach Q = 10 (we get 10 times more thermonuclear energy than we spend). This indicator is likely to be achieved on Tokamak (ITER) and Z-Machine, in the 2030s and later.
- Despite the achievement of Q = 10, thermonuclear reactors will be much more expensive than classical uranium because of the more complex design, shorter service life of the shell and superconducting magnets. Thermonuclear reactors also cannot be small (such as a floating mini-nuclear power plant)
- Not so much energy is released during a thermonuclear reaction - 11.5 times more energy is released per uranium fission than during D + T synthesis (which has the highest energy release among fusion reactions)
- Thermonuclear fuel is just not a lot - tritium is very expensive and scarce. Getting it is not easier and not cheaper than getting plutonium from uranium waste or U-233 from thorium.
- Helium-3 would not have helped mankind in any way, even if it were mountains on earth. The parasitic reaction of D + D will still produce radiation, and the optimum temperature will be a billion degrees, much more complicated than D + T over which humanity is beating at the moment.
- It looks like the next 1000 years we will use fast-neutron reactors, burn cheap uranium-238 and thorium (unless of course mankind destroys itself in another war)
- Nevertheless, humankind is obliged to continue working on thermonuclear energy, even if the commercial result will be in 1000 years, just as a thousand years ago scientists worked on the fundamentals of mathematics - without them there would be no current progress.